Plant Disease and Resistance
Broadly defined, disease is any physiological abnormality or significant disruption in the “normal” health of a plant. Disease can be caused by living (biotic) agents, including fungi and bacteria, or by environmental (abiotic) factors such as nutrient deficiency, drought, lack of oxygen, excessive temperature, ultraviolet radiation, or pollution. In order to protect themselves from damage, plants have developed a wide variety of constitutive and inducible defenses. Constitutive (continuous) defenses include many preformed barriers such as cell walls, waxy epidermal cuticles, and bark. These substances not only protect the plant from invasion, they also give the plant strength and rigidity. In addition to preformed barriers, virtually all living plant cells have the ability to detect invading pathogens and respond with inducible defenses including the production of toxic chemicals, pathogen-degrading enzymes, and deliberate cell suicide. Plants often wait until pathogens are detected before producing toxic chemicals or defense-related proteins because of the high energy costs and nutrient requirements associated with their production and maintenance.
Plant Pathogens: Sneak Attack versus Brute Force
Many plant pathogens act like “silent thieves” who want to steal money locked inside of a bank vault. These thieves use specialized tools designed to disable the bank’s security system and unlock the vault without being detected. In a similar way, many pathogens establish intimate connections with their hosts in order to suppress plant defenses and promote the release of nutrients. Pathogens that keep their host alive and feed on living plant tissue are called biotrophs. Examples of biotrophic pathogens include the powdery mildew fungus Blumeria graminis and the bacterial rice pathogen Xanthomonas oryzae. Other pathogens resort to brute force like thieves who blast open a bank vault with explosives. These pathogens often produce toxins or tissue-degrading enzymes that overwhelm plant defenses and promote the quick release of nutrients. These pathogens are called necrotrophs, and examples include the gray mold fungus Botrytis cinerea and the bacterial soft-rot pathogen Erwinia carotovora. Some pathogens are biotrophic during the early stages of infection but become necrotrophic during the latter stages of disease. These pathogens are called hemibiotrophs and include the fungus Magnaporthe grisea, the causative agent of rice blast disease.
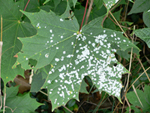 |
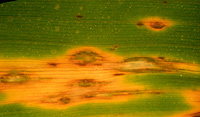 |
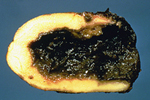 |
Figure 1. Powdery mildew on a maple leaf |
Figure 2. Rice blast disease on rice leaves |
Figure 3. Blackleg soft-rot on a potato tuber |
Most biotrophic and hemibiotrophic pathogens can only cause disease on a relatively small group of host plants because of the slightly different set of specialized genes and molecular mechanisms required for each host-pathogen interaction. The host range refers to the plant species on which a pathogen is capable of causing disease. For example, brome mosaic virus (BMV) infects grasses such as barley but not legumes. A plant species that does not show disease when infected with a pathogen is referred to as a non-host plant species for that pathogen. Organisms that do not cause disease on any plant species, such as the saprophytic bacterial species Pseudomonas putida, are referred to as non-pathogens.
When a pathogen is capable of causing disease on a particular host species, two outcomes are possible: A compatible response is an interaction that results in disease, while an incompatible response is an interaction that results in little or no disease at all. Although a particular plant species may be a susceptible host for a particular pathogen, some individuals may harbor genes that help recognize the presence of the pathogen and activate defenses. For example, some tomato cultivars show disease when infected with the bacterial pathogen Pseudomonas syringae (a compatible response), but others (cultivar Rio Grande, for example) are capable of recognizing the bacteria and limiting disease via resistance (an incompatible response). Disease resistance exists as a continuum of responses ranging from immunity (the complete lack of any disease symptoms) to highly resistant (some disease symptoms) to highly susceptible (significant disease symptoms). |
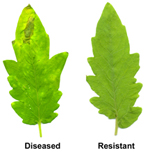 Figure 4. Tomato leaves exposed to the bacterial pathogen P. syringae. The leaf on the left is diseased, and the leaf on the right is resistant. |
Surveillance and Detection of Microbial Pathogens
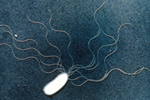 Figure 5. Bacterial flagella are often recognized by plants during basal resistance. |
Plants have developed multiple layers of sophisticated surveillance mechanisms that recognize potentially dangerous pathogens and rapidly respond before those organisms have a chance to cause serious damage. These surveillance systems are linked to specific pre-programmed defense responses. Basal resistance, also called innate immunity, is the first line of pre-formed and inducible defenses that protect plants against entire groups of pathogens. Basal resistance can be triggered when plant cells recognize microbe-associated molecular patterns (MAMPs) including specific proteins, lipopolysaccharides, and cell wall components commonly found in microbes. The result is that living plant cells become fortified against attack. Non-pathogens as well as pathogens are capable of triggering basal resistance in plants due to the widespread presence of these molecular components in their cells. |
Pathogens have developed countermeasures that are able to suppress basal resistance in certain plant species. If a pathogen is capable of suppressing basal defense, plants may respond with another line of defense: the hypersensitive response (HR). The HR is characterized by deliberate plant cell suicide at the site of infection. Although drastic compared to basal resistance, the HR may limit pathogen access to water and nutrients by sacrificing a few cells in order to save the rest of the plant. The HR is typically more pathogen-specific than basal resistance and is often triggered when gene products in the plant cell recognize the presence of specific disease-causing effector molecules introduced into the host by the pathogen. Bacteria, fungi, viruses, and microscopic worms called nematodes are capable of inducing the HR in plants. |
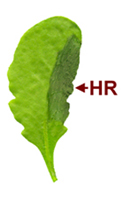 Figure 6. HR lesion on an Arabidopsis leaf. |
Once the hypersensitive response has been triggered, plant tissues may become highly resistant to a broad range of pathogens for an extended period of time. This phenomenon is called systemic acquired resistance (SAR) and represents a heightened state of readiness in which plant resources are mobilized in case of further attack. Researchers have learned to artificially trigger SAR by spraying plants with chemicals called plant activators. These substances are gaining favor in the agricultural community because they are much less toxic to humans and wildlife than fungicides or antibiotics, and their protective effects can last much longer.
In addition to the hypersensitive response, plants can defend themselves against viruses by a variety of mechanisms including a sophisticated genetic defense system called RNA silencing. Many viruses produce double-stranded RNA or DNA during replication in a host cell. Plants can recognize these foreign molecules and respond by digesting the genetic strands into useless fragments and halting the infection. Plants that are infected with viruses will often exhibit chlorosis and mottling, but disease symptoms may eventually disappear if RNA silencing is successful, a process called recovery. In addition, the plant may retain a template of the digested genetic strand that can be used to quickly respond to future attack by similar viruses, a process analogous to the memory of vertebrate immune systems.
Detection of Insect Herbivores
Mechanical damage caused by insects is not generally considered “true” plant disease although plants have developed surveillance systems designed to recognize insect pests and respond with specific defense mechanisms. Plants can distinguish between general wounding and insect feeding by the presence of elicitors contained in the saliva of chewing insects. In response, plants may release volatile organic compounds (VOCs), including monoterpenoids, sesquiterpenoids, and homoterpenoids. These chemicals may repel harmful insects or attract beneficial predators that prey on the destructive pests. For example, wheat seedlings infested with aphids may produce VOCs that repel other aphids. Lima beans and apple trees emit chemicals that attract predatory mites when damaged by spider mites, and cotton plants produce volatiles that attract predatory wasps when damaged by moth larvae. Feeding on one part of the plant can induce systemic production of these chemicals in undamaged plant tissues, and once released, these chemicals can act as signals to neighboring plants to begin producing similar compounds. Production of these chemicals exacts a high metabolic cost on the host plant, so many of these compounds are not produced in large quantities until after insects have begun to feed.
Structural Defenses
The Plant Cell
All plant tissues contain pre-formed structural barriers that help limit pathogen attachment, invasion and infection. The cell wall is a major line of defense against fungal and bacterial pathogens. It provides an excellent structural barrier that also incorporates a wide variety of chemical defenses that can be rapidly activated when the cell detects the presence of potential pathogens. All plant cells have a primary cell wall, which provides structural support and is essential for turgor pressure, and many also form a secondary cell wall that develops inside of the primary cell wall after the cell stops growing. The primary cell wall consists mostly of cellulose, a complex polysaccharide consisting of thousands of glucose monomers linked together to form long polymer chains. These chains are bundled into fibers called microfibrils, which give strength and flexibility to the wall. The cell wall may also contain two groups of branched polysaccharides: cross-linking glycans and pectins. Cross-linking glycans include hemicellulose fibers that give the wall strength via cross-linkages with cellulose. Pectins form hydrated gels that help “cement” neighboring cells together and regulate the water content of the wall. Soft-rot pathogens often target pectins for digestion using specialized enzymes that cause cells to break apart: these organisms are extremely common, and anyone who has seen fruits or vegetables become brown and “mushy” have seen these pathogens in action. |
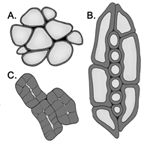 Figure 7. Comparison of cell wall types. The primary cell walls of red pepper cells (A) are relatively thin compared to the thick secondary cell walls of oak wood (B). The walls of pear fruit stone cells (C) are so thick that the cell lumen is barely visible. |
Many cell walls also contain lignin, a heterogeneous polymer composed of phenolic compounds that gives the cell rigidity. Lignin is the primary component of wood, and cell walls that become “lignified” are highly impermeable to pathogens and difficult for small insects to chew. Cutin, suberin, and waxes are fatty substances that may be deposited in either primary or secondary cell walls (or both) and outer protective tissues of the plant body, including bark.
Cell walls contain proteins and enzymes that actively work to reshape the wall during cell growth yet thicken and strengthen the wall during induced defense. When a plant cell detects the presence of a potential pathogen, enzymes catalyze an oxidative burst that produces highly reactive oxygen molecules capable of damaging the cells of invading organisms. Reactive oxygen molecules also help strengthen the cell wall by catalyzing cross-linkages between cell wall polymers, and they serve as a signal to neighboring cells that an attack is underway. Plant cells also respond to microbial attack by rapidly synthesizing and depositing callose between the cell wall and cell membrane adjacent to the invading pathogen. Callose deposits, called papillae, are polysaccharide polymers that impede cellular penetration at the site of infection, and these are often produced as part of the induced basal defense response.
Some plant cells are highly specialized for plant defense. Idioblasts (“crazy cells”) help protect plants against herbivory because they contain toxic chemicals or sharp crystals that tear the mouthparts of insects and mammals as they feed. There are many classes of idioblasts including pigmented cells, sclereids, crystalliferous cells, and silica cells. Pigmented cells often contain bitter-tasting tannins that make plant parts undesirable as a food source. Young red wines often contain high levels of tannins that give wine a sharp, biting taste. Sclereids are irregularly-shaped cells with thick secondary walls that are difficult to chew: the rough texture of pear fruit (Pyrus spp.) is caused by thousands of sclereid stone cells that can abrasively wear down the teeth of feeding animals. Stinging nettles (Urtica dioica) produce stinging cells shaped like hypodermic needles that break off when disturbed and inject highly irritating toxins into herbivore tissues. Some stinging cells contain prostaglandins, hormones that amplify pain receptors in vertebrate animals and increase the sensation of pain. Crystalliferous cells contain crystals of calcium oxalate that may tear herbivore mouthparts when chewed and can be toxic if ingested. Members of the genera Philodendron and Dieffenbachia are very common tropical house plants that contain large amounts of these cells. Humans and pets who chew the leaves of these plants may experience a burning sensation in the mouth and throat that is often accompanied by swelling, choking, and an inability to speak. For these reasons, species of Dieffenbachia are commonly called dumb cane. Grasses and sedges contain rows of silica cells in their epidermal layers which give strength and rigidity to the growing leaf blades and deter feeding by chewing insects.
Plant Tissues and Specialized Appendages
The epidermis constitutes the outermost protective tissue system of leaves, floral parts, fruits, seeds, stems, and roots of plants until they undergo considerable secondary growth. It is the first line of defense against invading pathogens and consists of both specialized and unspecialized cells. The epidermal cells of aerial plant parts are often covered in a waxy cuticle that not only prevents water loss from the plant, but also prevents microbial pathogens from coming into direct contact with epidermal cells and thereby limits infection. The cuticle can be relatively thin (aquatic plants) or extremely thick (cacti). The hydrophobic nature of the cuticle also prevents water from collecting on the leaf surface, an important defense against many fungal pathogens that require standing water on the leaf surface for spore germination. However, some fungal pathogens including Fusarium solani produce cutinases that degrade the cuticle and allow the fungi to penetrate the epidermis. |
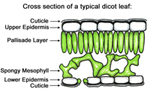 Figure 8. The epidermis is a protective layer of cells that is usually covered with a thin, water-resistant cuticle that helps prevent water loss from the plant. |
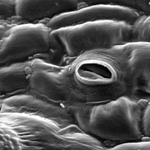 Figure 9. Stomate on the surface of a tomato leaf. Note the small rod-shaped bacteria. |
Interspersed among the many unspecialized cells of the epidermis are guard cells which regulate gas exchange through small openings called stomata. These pores allow carbon dioxide to enter the leaf for use in photosynthesis while restricting excessive water loss from the plant. Stomatal pore size is highly regulated by plants, and guard cells can participate in defense by closing in response to the presence of MAMPs. |
Trichomes (“leaf hairs”) are specialized epidermal cells found on aerial plant parts that may provide both physical and chemical protection against insect pests. The velvety appearance of dusty miller (Senecio cineraria) is caused by thousands of tiny trichomes covering the plant’s surface. Trichomes on the surface of soybeans (Glycine max) prevent insect eggs from reaching the epidermis and the larvae starve after hatching. The hook-shape of snap bean (Phaseolis vulgaris) trichomes impale caterpillars as they move across the leaf surface, and glandular trichomes in potato and tomato secrete oils that repel aphids. In woody plants, the periderm replaces the epidermis on stems and roots. Outer bark (phellem) is an excellent example of a preformed structural barrier that contains high amounts of water-resistant suberin and prevents many pathogens and insects from reaching the living cells underneath. |
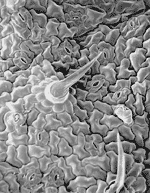 Figure 10. Phaseolis vulgaris bean leaf surface with stomata and uniseriate trichomes. |
Thorns are modified branches that protect plants from grazing vertebrates, and include the honey locust tree (Gleditsia triacanthos). Many cacti produce thorn-like structures that are actually modified leaves or parts of leaves (e.g., stipules) called spines which serve similar purposes, such as in the barrel cactus (Ferocactus spp.). Botanically speaking, the “thorns” on the stem of rose plants (Rosa spp.) are neither true thorns nor spines: they are actually outgrowths of the epidermis called prickles.
Chemical Defenses
Plant chemicals can be divided into two major categories: primary metabolites and secondary metabolites. Primary metabolites are substances produced by all plant cells that are directly involved in growth, development, or reproduction. Examples include sugars, proteins, amino acids, and nucleic acids. Secondary metabolites are not directly involved in growth or reproduction but they are often involved with plant defense. These compounds usually belong to one of three large chemical classes: terpenoids, phenolics, and alkaloids.
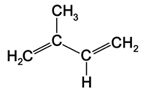 Figure 11. Isoprene |
Terpenoids (terpenes) occur in all plants and represent the largest class of secondary metabolites with over 22,000 compounds described. The simplest terpenoid is the hydrocarbon isoprene (C5H8), a volatile gas emitted during photosynthesis in large quantities by leaves that may protect cell membranes from damage caused by high temperature or light. Terpenoids are classified by the number of isoprene units used to construct them. For example, monoterpenoids consist of two isoprene units, sesquiterpenoids (three units), diterpenoids (four units), and triterpenoids (six units). |
Monoterpenoids and sesquiterpenoids are the primary components of essential oils, which are highly volatile compounds that contribute to the fragrance (essence) of plants that produce them. Essential oils often function as insect toxins and many protect against fungal or bacterial attack. Mint plants (Mentha spp.) produce large quantities of the monoterpenoids menthol and menthone which are produced and stored in glandular trichomes on the epidermis. Pyrethrins are monoterpenoid esters produced by chrysanthemum plants that act as insect neurotoxins. Many commercially available insecticides are actually synthetic analogues of pyrethrins, called pyrethroids, including the insecticides permethrin and cypermethrin. Pine tree resin contains large quantities of the monoterpenoids alpha- and beta-pinene, which are potent insect repellents; these compounds give the organic solvent turpentine its characteristic sharp odor. |
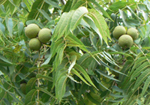 Figure 12. Black walnuts (Juglans nigra) produce juglone, a chemical that interferes with the normal development of other plants, especially members of the nightshade family (e.g., tomato). |
Monoterpenoids are not just used as insecticides. Many spices, seasonings, condiments, and perfumes are made using essential oils that function as insect toxins in plants but are relatively harmless to humans. Examples include peppermint and spearmint (Mentha spp.), basil (Ocimum spp.), oregano (Origanum spp.), rosemary (Rosmarinus spp.), sage (Salvia spp.), savory (Satureja spp.), thyme (Thymus spp.), black pepper (Piper spp.), cinnamon (Cinnamomum spp.), and bay leaf (Laurus spp.).
Diterpenoids include gossypol, a terpenoid produced by cotton (Gossypium hirsutum) that has strong antifungal and antibacterial properties. Triterpenoids are similar in molecular structure to plant and animal sterols and steroid hormones. Phytoectysones are mimics of insect molting hormones. When produced by plants such as spinach (Spinacia oleracea), they disrupt larval development and increase insect mortality. The fresh scent of lemon and orange peels is the result of a class of triterpenoids called limonoids. Azadirachtin is a very powerful limonoid isolated from neem trees (Azadirachta indica): some insects are repelled by concentrations as low as a few parts per million. Citronella is an essential oil isolated from lemon grass (Cymbopogon citratus); it contains high limonoid levels and has become a popular insect repellent in the United States due to its low toxicity in humans and biodegradable properties.
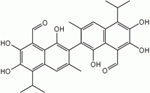 |
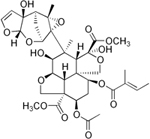 |
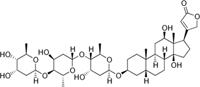 |
Figure 13. Gossypol |
Figure 14. Azadirachtin |
Figure 15. Digoxin |
Insects are not the only herbivores that feed on plants. Triterpenoids such as cardiac glycosides are highly toxic to vertebrate herbivores, including humans, and can cause heart attacks if ingested in high quantities. Foxglove (Digitalis purpurea) is the principal source of the cardiac glycosides digitoxin and digoxin, which are used medicinally in small quantities to treat heart disease in people. Some herbivores have overcome the dangerous effects of cardiac glycosides and actually use these toxins for their own benefit. Monarch butterfly caterpillars feed almost exclusively upon milkweed (Asclepias spp.) which contains high amounts of these toxins in the milky latex of their sap. The caterpillars store these toxins safely within their bodies, and when the caterpillars become adult butterflies, they are highly poisonous to most predatory birds that eat them. |
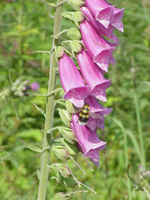 Figure 16. Digitalis purpurea |
Saponins are glycosylated triterpenoids (triterpenoids with attached sugar groups) that are present in the cell membranes of many plant species. These substances have detergent (soap-like) properties and disrupt the cell membranes of invading fungal pathogens. The wheat pathogen Gaeumannomyces graminis is unable to infect oats that contain avenacins, a class of triterpenoid saponins. However, some fungal pathogens have developed counter-measures to these plant defenses: Botrytis cinerea, Fusarium oxysporum, and Septoria lycopersici are all capable of degrading saponins and causing disease in susceptible saponin-producing plants.
Phenolics
Phenolics are another large class of secondary metabolites produced by plants to defend themselves against pathogens. They are produced primarily via the shikimic acid and malonic acid pathways in plants, and include a wide variety of defense-related compounds including flavonoids, anthocyanins, phytoalexins, tannins, lignin, and furanocoumarins. Flavonoids are one of the largest classes of phenolics. Anthocyanins are colorful water-soluble flavonoids pigments produced by plants to protect foliage from the damaging effects of ultraviolet radiation. Anthocyanins are responsible for the showy colors of many plants and are present in high concentrations in flowers, fruits, and the leaves of deciduous plants in fall. Phytoalexins are isoflavonoids with antibiotic and antifungal properties that are produced in response to pathogen attack. These toxic molecules disrupt pathogen metabolism or cellular structure but are often pathogen specific in their toxicity. Examples include medicarpin produced by alfalfa (Medicago sativa), rishitin produced by both tomatoes and potatoes (the Solanaceae family), and camalexin, produced by Arabidopsis thaliana.
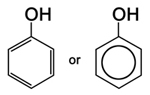 |
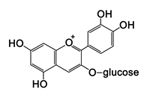 |
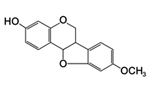 |
Figure 17. Phenol, the simplest phenolic compound |
Figure 18. Cyanin glycoside, an anthocyanin |
Figure 19. Medicarpin, a phytoalexin |
Tannins are water-soluble flavonoid polymers produced by plants and stored in vacuoles. Tannins are toxic to insects because they bind to salivary proteins and digestive enzymes including trypsin and chymotrypsin resulting in protein inactivation. Insect herbivores that ingest high amounts of tannins fail to gain weight and may eventually die. The sharp taste of red wine is caused by grape tannins binding to salivary proteins in the mouth which results in protein coagulation.
Lignin is a highly branched heterogeneous polymer found principally in the secondary cell walls of plants, although primary walls can also become lignified. It consists of hundreds or thousands of phenolic monomers and is a primary component of wood. Because it is insoluble, rigid, and virtually indigestible, lignin provides an excellent physical barrier against pathogen attack.
Furanocoumarins are phenolic compounds produced by a wide variety of plants in response to pathogen or herbivore attack. They are activated by ultraviolet light and can be highly toxic to certain vertebrate and invertebrate herbivores due to their integration into DNA, which contributes to rapid cell death. In fact, grapefruit juice contains small quantities of furanocoumarins, which greatly increase the absorption of certain drugs into the bloodstream from the intestines. Some medicines carry warning labels cautioning people to avoid drinking grapefruit juice while taking the drugs in order to avoid an accidental overdose. |
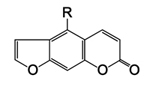 Figure 20. Psoralen, a furanocoumarin |
Nitrogen Compounds
Alkaloids are a large class of bitter-tasting nitrogenous compounds that are found in many vascular plants and include caffeine, cocaine, morphine, and nicotine. They are derived from the amino acids aspartate, lysine, tyrosine, and tryptophan, and many of these substances have powerful effects on animal physiology. Caffeine is an alkaloid found in plants such as coffee (Coffea arabica), tea (Camellia sinensis), and cocoa (Theobroma cacao). It is toxic to both insects and fungi. In fact, high levels of caffeine produced by coffee seedlings can even inhibit the germination of other seeds in the vicinity of the growing plants, a phenomenon called allelopathy. Allelopathy allows one plant species to “defend” itself against other plants that may compete for growing space and nutrient resources.
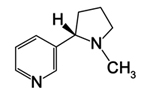 |
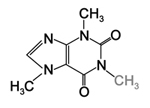 |
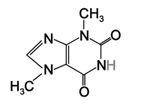 |
Figure 21. Nicotine |
Figure 22. Caffeine |
Figure 23. Theobromine |
Members of the nightshade family (Solanaceae) produce many important alkaloid compounds. Nicotine is an alkaloid that is produced in the roots of tobacco plants (Nicotiana tabacum) and transported to leaves where it is stored in vacuoles. It is released when herbivores graze on the leaves and break open the vacuoles. Atropine is a neurotoxin and cardiac stimulant produced by the deadly nightshade plant (Atropa belladonna). Although it is toxic in large quantities, it has been used medicinally by humans in small amounts as a pupil dilator and antidote for some nerve gas poisonings. Capsaicin and related capsaicinoids produced by members of the genus Capsicum are the active components of chili peppers and produce their characteristic burning sensation in hot, spicy foods.
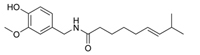 |
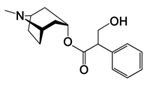 |
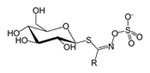 |
Figure 24. Capsaicin |
Figure 25. Atropine |
Figure 26. Glucosinolate |
Cyanogenic glycosides are a particularly toxic class of nitrogenous compounds that break down to produce hydrogen cyanide (HCN), a lethal chemical that halts cellular respiration in aerobic organisms. Plants that produce cyanogenic glycosides also produce enzymes that convert these compounds into hydrogen cyanide, including glycosidases and hydroxynitrile lyases, but they are stored in separate compartments or tissues within the plant; when herbivores feed on these tissues, the enzymes and substrates mix and produce lethal hydrogen cyanide. Glucosinolates, also known as mustard oil glycosides, are sulfur-containing compounds synthesized by members of the mustard family (Brassicaceae) and produce cyanide gas when broken down by enzymes called thioglucosidases.
Proteins and Enzymes
Many plants and seeds contain proteins that specifically inhibit pathogen and pest enzymes by forming complexes that block active sites or alter enzyme conformations, ultimately reducing enzyme function. These proteins are generally small and rich in the amino acid cysteine. They include defensins, amylase inhibitors, lectins, and proteinase inhibitors. Unlike simple chemicals such as terpenoids, phenolics, and alkaloids, proteins require a great deal of plant resources and energy to produce; consequently, many defensive proteins are only made in significant quantities after a pathogen or pest has attacked the plant. Once activated, however, defensive proteins and enzymes effectively inhibit fungi, bacteria, nematodes, and insect herbivores.
Defensins are small cysteine-rich proteins that display broad anti-microbial activity and were first isolated from the endosperm of barley (Hordeum vulgare) and wheat (Triticum aestivum). They are widely distributed and may be present in most plants. Defensins are best characterized in seeds, but can be found in virtually all types of plant tissues including leaves, pods, tubers, fruit, roots, bark, and floral tissues. They exhibit a wide range of biological activities that serve to inhibit the growth of many fungi and bacteria. Some defensins also inhibit digestive proteins in herbivores. The precise mechanisms employed by plant defensins to inhibit fungi and bacteria are still being characterized, but they appear to act upon molecular targets in the plasma membrane of pathogens. These defensins may inhibit pre-existing ion channels or form new membrane pores that disrupt cellular ion balance. |
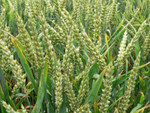 Figure 27. Wheat (Triticum aestivum) |
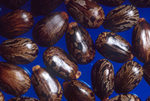 Figure 28. Castor beans (Ricinus communis) |
Digestive enzyme inhibitors are proteins that block the normal digestion and absorption of nutrients by vertebrate and invertebrate herbivores. Alpha-amylase inhibitors are proteins commonly found in legumes that bind to amylase enzymes and inhibit starch digestion. Lectins are non-enzymatic proteins and glycoproteins that bind to carbohydrates and exhibit a wide range of functions including disruption of digestion in insects and agglutination of blood cells in vertebrates. Ricin is a powerful toxin produced in castor beans (Ricinus communis). It combines a lectin molecule with an N-glycoside hydrolase that enters animal cells and inhibits protein synthesis. Ricin is a highly potent toxin, having an average lethal dose of only 0.2 milligrams in humans. |
Protease inhibitors are typically produced in response to herbivore attack and inhibit digestive enzymes including trypsin and chymotrypsin. They occur widely in nature but have been well studied in legumes, solanaceous plants, and grasses. Herbivore feeding often triggers a series of molecular signaling events that induce systemic production of these compounds in distal tissues that contribute to the protection of undamaged plant parts from subsequent attacks by a wide range of herbivore pests.
Hydrolytic enzymes are produced by some plants in response to pathogens and often accumulate in extracellular spaces where they degrade the cell walls of pathogenic fungi. Chitinases are enzymes that catalyze the degradation of chitin, a polymer with a backbone similar to cellulose that is present in the cell walls of true fungi. Glucanases are enzymes that catalyze the degradation of glycosidic linkages in glucans, a class of polymers similar to cellulose that is present in the cell walls of many oomycetes (water molds). In vitro analysis has verified the anti-fungal properties of these compounds, and transgenic plants expressing high levels of these enzymes exhibit increased resistance to a wide range of both foliar and root pathogens. Lysozymes are hydrolytic enzymes that are capable of degrading bacterial cell walls.
Additional Plant Defenses
The mechanisms discussed in this article represent a broad overview of plant defense responses. However, the reader is encouraged to investigate other aspects of plant defense including symbiotic relationships, the importance of beneficial microbes on plant health, and the impact of environmental conditions on plant disease. One may also want to learn more about how humans influence disease resistance, including integrated pest management strategies, biological control, cultural practices, and genetic engineering.
Suggested Readings
Evert, R.F., and S.E. Eichhorn. 2006. Esau's Plant Anatomy: Meristems, Cells, and Tissues of the Plant Body: Their Structure, Function, and Development. John Wiley & Sons, Hoboken, NJ.
Agrawal, A., S. Tuzun, and E. Bent. 1999. Induced Plant Defenses Against Pathogens and Herbivores: Biochemistry, Ecology, and Agriculture. APS Press Inc., Saint Paul, MN.
References
- Albert, M., X. Belastegui-Macadam, and R. Kaldenhoff. 2006. An attack of the plant parasite Cuscuta reflexa induces the expression of attAGP, an attachment protein of the host tomato. Plant Journal 48(4):548-556.
- Bindschedler L.V., J. Dewdney, K.A. Blee, J.M. Stone, T. Asai, J. Plotnikov, C. Denoux, T. Hayes, C. Gerrish, D.R. Davies, F.M. Ausubel, and G.P. Bolwell. 2006. Peroxidase-dependent apoplastic oxidative burst in Arabidopsis required for pathogen resistance. Plant Journal 47(6):851-863.
- Broekaert, W.F., B.P.A. Cammue, M.F.C. DeBolle, K. Thevissen, G. W. DeSamblanx, and R.W. Osborne. 1997. Antimicrobial peptides from plants. Critical Reviews in Plant Sciences 16(3):297-323.
- Broekaert, W.F., F.R. Terras, B.P. Cammue, and R.W. Osborn. 1995. Plant defensins - Novel antimicrobial peptides as components of the host-defense system. Plant Physiology 108(4):1353-1358.
- Dow, M., M.A. Newman, and E. von Roepenack. 2000. The induction and modulation of plant defense responses by bacterial lipopolysaccharides. Annual Review of Phytopathology 38:241-261.
- Gilchrist, D.G. 1998. Programmed cell death in plant disease: the purpose and promise of cellular suicide. Annual Review of Phytopathology 36:393-414.
- Grennan, A.K. 2006. Plant response to bacterial pathogens. Overlap between innate and gene-for-gene defense response. Plant Physiology 142(3):809-811.
- Ham, J.H., M.G. Kim, S.Y. Lee, and D. Mackey. 2007. Layered basal defenses underlie non-host resistance of Arabidopsis to Pseudomonas syringae pv. phaseolicola. Plant Journal 51(4):604-616.
- Heath, M.C. 2000. Hypersensitive response-related death. Plant Molecular Biology 44(3):321-334.
- Heil, M., and R.M. Bostock. 2002. Induced systemic resistance (ISR) against pathogens in the context of induced plant defenses. Annals of Botany (London), 89(5):503-512.
- Jacobs, A.K., V. Lipka, R.A. Burton, R. Panstruga, N. Strizhov, P. Schulze-Lefert, and G.B. Fincher. 2003. An Arabidopsis callose synthase, GSL5, Is required for wound and papillary callose formation. Plant Cell 15(11):2503-2513.
- Lay, F.T., and M.A. Anderson. 2005. Defensins-components of the innate immune system in plants. Current Protein Peptide Science 6(1):85-101.
- Lee, S., H. Choi, S. Suh, I. Doo, K. Oh, E.J. Choi, A.T. Schroeder-Taylor, P.S. Low, and Y. Lee. 1999. Oligogalacturonic acid and chitosan reduce stomatal aperture by inducing the evolution of reactive oxygen species from guard cells of tomato and Commelina communis. Plant Physiology 121(1):147-152.
- Liu, Y., M. Schiff, K. Czymmek, Z. Tallóczy, B. Levine, and S. Dinesh-Kumar. 2005. Autophagy regulates programmed cell death during the plant innate immune response. Cell 121(4):567-577.
- McCloud, E.S., and I.T. Baldwin. 1997. Herbivory and caterpillar regurgitants amplify the wound-induced increases in jasmonic acid but not nicotine in Nicotiana sylvestris. Planta 203(4):430-435.
- Mysore, K.S., and C.M. Ryu. 2004. Nonhost resistance: how much do we know? Trends in Plant Science 9(2):97-104.
- Nurnberger, T., F. Brunner, B. Kemmerling, and L. Piater. 2004. Innate immunity in plants and animals: striking similarities and obvious differences. Immunological Reviews 198(1):249-266.
- Oh, H.S. and A. Collmer. 2005. Basal resistance against bacteria in Nicotiana benthamiana leaves is accompanied by reduced vascular staining and suppressed by multiple Pseudomonas syringae type III secretion system effector proteins. Plant Journal 44(2):348-359.
- Osborn, R.W., G.W. Desamblanx, K. Thevissen, I. Goderis, S. Torrekens, F. Vanleuven, S. Attenborough, S. Rees, and W.F. Broekaert. 1995. Isolation and characterization of plant defensins from seeds of Asteraceae, Fabaceae, Hippocastanaceae and Saxifragaceae. FEBS Letters 368(2):257-262.
- Pare, P.W., and J.H. Tumlinson. 1999. Plant volatiles as a defense against insect herbivores. Plant Physiology 121(2):325-331.
- Quiroz, A., J. Pettersson, J. A. Pickett, L. J. Wadhams, and H. M. Niemeyer. 1997. Semiochemicals mediating spacing behavior of bird cherry-oat aphid, Rhopalosiphum padi feeding on cereals. Journal of Chemical Ecology 23(11):2599-2607.
- Rose, U.S.R., A. Manukian, R.R. Heath, and J.H. Tumlinson. 1996. Volatile semiochemicals released from undamaged cotton leaves - A systemic response of living plants to caterpillar damage. Plant Physiology 111(2):487-495.
- Shah, J. 2005. Lipids, lipases, and lipid-modifying enzymes in plant disease resistance. Annual Review of Phytopathology 43:229-260.
- Takabayashi, J., and M. Dicke. 1996. Plant-carnivore mutualism through herbivore-induced carnivore attractants. Trends in Plant Science 1(4):109-113.
- Tumlinson, J.H., W.J. Lewis, and L.E.M. Vet. 1993. How parasitic wasps find their hosts. Scientific American 268(3):100-106.
- van Loon, L.C., M. Rep, and C.M. Pieterse. 2006. Significance of inducible defense-related proteins in infected plants. Annual Review of Phytopathology 44:135-162.
- Veronese, P., M. T. Ruiz, M. A. Coca, A. Hernandez-Lopez, H. Lee, J.I. Ibeas, B. Damsz, J.M. Pardo, P.M. Hasegawa, R.A. Bressan, and M.L. Narasimhan. 2003. In defense against pathogens: Both plant sentinels and foot soldiers need to know the enemy. Plant Physiology 131(4):1580-1590.
- Zhang, C., A.T. Gutsche, and A.D. Shapiro. 2004. Feedback control of the Arabidopsis hypersensitive response. Molecular Plant-Microbe Interaction 17(4):357-365.
- Zipfel, C., S. Robatzek, L. Navarro, E.J. Oakeley, J.D.G. Jones, G. Felix, and T. Boller. 2004. Bacterial disease resistance in Arabidopsis through flagellin perception. Nature 428(6984):764-767.