Martyn, R.D. 2002. Monosporascus root rot and vine decline of melons. The Plant Health Instructor. DOI: 10.1094/PHI-I-2002-0612-01
Updated 2009.
Monosporascus Root Rot and Vine Decline of Melons (MRR/VD). Also referred to as sudden wilt, sudden death, melon collapse, Monosporascus wilt, and black pepper root rot.
Monosporascus cannonballus Pollack & Uecker
Melon (Cucumis melo) and watermelon (Citrullus lanatus (Thunb.) Matsum & Nakai) are the principal economic hosts. Most other cucurbits, including cucumber (Cucumis sativus), bottle gourd (Lagenaria siceraria), and Cucurbita spp., are susceptible to artificial inoculation but are rarely observed infected under commercial field conditions. Infection has been observed, however, in several cucurbit species in commercial greenhouse production. Other noncucurbit plants are reported to support pathogen growth under artificial inoculation conditions or in the field without obvious symptoms of disease.
Author
Ray D. Martyn,
Purdue University
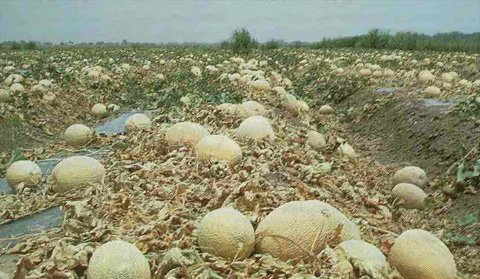
Collapse of melon plants due to M. cannonballus. (Courtesy R. Martyn)
Symptoms and Signs
Monosporascus cannonballus infects the roots of plants, primarily the secondary and tertiary roots. Infection can occur very early in the growing season, but above ground symptoms are not usually apparent until much later in the season. The primary symptoms are necrosis of the small roots and lesions on the larger roots, typically around the root junctions (Figure1), however the most dramatic symptom is the collapse of the vine late in the season (Figure 2).
 Figure 1 |
 Figure 2 |
Root colonization and necrosis continues throughout the life of the plant causing increasingly more damage to the root system. In severe cases, larger roots, including the taproot, become infected. The above ground symptoms displayed as a result of root damage are early season stunting of the plant and a gradual death of the leaves, beginning with the crown leaves. Typically, late in the season, the entire remaining vine collapses (Figure 2). Disease severity is exacerbated by plant stresses, including plant maturity, fruit load, and biotic and abiotic factors, including other soil microorganisms, soil temperature, moisture, and pH. Diseased plants have few secondary and tertiary roots (Figure 1) and may or may not display symptoms of a wet root rot where the cortex of the roots easily sloughs off. There is no indication that wet rot is due to M. cannonballus per se, but rather is most probably the result of secondary fungi or bacteria present in the soil taking advantage of a compromised root and moist soil conditions.
The primary diagnostic sign of the pathogen is the formation of black, spherical, erumpent perithecia on the roots (Figure 3), easily visible with the unaided eye or hand lens. Perithecia are the sexual reproductive structures (fruiting bodies) of the fungus and develop along the entire root length and, at times, can be quite numerous. They are most commonly observed on secondary lateral roots late in the season; however, they typically are more abundant after the plant (root) has been dead for a few days. This may result in the misdiagnosis of the disease or a very late diagnosis, often after the plant has died. Perithecia may be difficult to observe in the field because many of the necrotic roots bearing them are lost prior to or during extraction from the soil. When mature, the perithecia rupture, spilling the ascospores into the soil (Figures 4 & 5).
 Figure 3 |
 Figure 4 |
 Figure 5 |
Pathogen Biology
Monosporascus cannonballus is a pyrenomycete (Ascomycota: Sordariales) fungus, although the exact taxonomic placement probably needs re-examination. It forms large (40-50 µm-diam), spherical, multi-layer walled ascospores (Figures 6 & 7), however typically only one ascospore is formed per ascus instead of the usual eight (Figure 8). Ascospores are multinucleate, typically having eight nuclei, although occasionally they may have 16. There is no known anamorph (asexual) stage. Four species of Monosporascus have been described, but only two, M. cannonballus and M. eutypoides, have been implicated in disease of cucurbits. Both are pathogenic to melons and they were originally distinguished from each other on the basis of the number of ascospores per ascus and whether or not they germinated readily. Studies based on partial DNA sequence similarity, pathogenicity and other phenotypic traits, suggested that they are conspecific (the same species). More recent data on how the ascospores germinate and the number of germ tubes formed argue for maintaining them as separate species. However, isolates of M. eutypoides are difficult to locate making direct comparisons difficult. Since M. cannonballus is the original species name (holotype) and it is the major pathogen of vine decline reported around the world, its name has been retained in this paper.
 Figure 6 |
 Figure 7 |
 Figure 8 |
The fungus is homothallic (self-fertile) and readily forms fertile perithecia in host root tissue (Figures 3 and 4)and in vitro on artificial growth media (Figure 5). Ascospores are thick, multi-walled spores (Figures 6, 7, and 8) and are extremely resistant to desiccation and other factors. Germination of the ascospore is rare in vitro, however germination is enhanced in situ when in the presence of root exudates from growing muskmelon seedlings. Soil microflora, most likely actinomycetes also are important in the germination of ascospores in the field.Monosporascus cannonballus is adapted to hot, dry climates.In vitro vegetative growth is optimal at 25 to 35°C (77 to 95°F), while perithecia are formed most readily at 25 to 30°C (77 to 86°F). The fungus may survive for several days at temperatures up to 55 C°, but is killed within 90 min at 60°C. Mycelial growth occurs over a pH range of 5 to 9, but is optimal from pH 6 to 7 and inhibited completely at pH 4 and below.Monosporascus cannonballus appears also to be adapted to slightly or moderately alkaline and saline soils. The fungus grows readily on several standard laboratory growth media (e.g. potato dextrose agar, V-8 juice agar, and water agar) and forms fertile black perithecia within 2 to 3 weeks. Perithecia are readily visible against the light gray or dirty white mycelium.
In culture (e.g., PDA, V-8 juice agar, water agar), wild-type Monosporascus colonies typically are a dirty-white or light grey color with numerous black perithecia embedded in the agar. Under some circumstances, colonies may produce pigments giving the mycelia a yellow to orange to dark brown color. Pigmented colonies are slow-growing, produce few, if any, perithecia, and are often hypovirulent. Colony death often occurs in heavily pigmented cultures. Five different hexaketides have been identified from pigmented cultures. Little is known as to why some colonies develop these dark pigments but dsRNA may play a role. dsRNA also may play a role in decreased perithecia production and hypovirulence.
There appears to be little genetic variation within M. cannonballus. Although a detailed analysis of a large population has not been done, selected isolates of M. cannonballus from several countries, including the U. S., Japan, and Spain, were identical in colony morphology, number of ascospores/ascus (one), virulence to melon, and DNA sequence across the internal transcribed spacer regions (ITS 1 and 2) and 5.8S gene of the rDNA, suggesting that most isolates are genetically very similar. There do appear to be some differences in vegetative compatibility among U.S. isolates and those from other regions of the world. This aspect has not been studied in depth and could add valuable information to understanding the genetics of this fungus.
In some instances, extreme variations in colony appearance, including the formation of dark yellow, orange or brown pigments (Figure 14), reduced vegetative growth, and loss of perithecia production, as well as loss of virulence and colony death, have been reported. Isolates displaying one or more of these phenotypes harbored one or more double-stranded RNAs (dsRNA), which were attributed to causing the aberrant phenotype. Several distinct dsRNA groupings have been identified. This has been explored as a potential biocontrol of MRR/VD.
 Figure 14 |
Disease Cycle
Disease cycle of Monosporascus root rot and vine decline.
A) Mature ascospores in soil; B) Germinated ascopsore in the rhizosphere and attachment to the root; C) Necrotic lesions on melon roots; D) Above ground symptoms of vine decline and death; E) Perithecia formed in infected root tissue; F) Mature perithecium releasing ascospores into soil. (Courtesy R. Cohen)
The disease cycle of M. cannonballus appears to be relatively simple. Infection of the roots can occur from either mycelium that has survived in the soil or plant debris or from germinating ascospores (A-B in disease cycle & Figure 10). The fungus invades the fine feeder roots (B-C in disease cycle & Figure 11) colonizes the cortex and xylem, and kills the rootlet.
 Figure 10 |
 Figure 11 |
 Figure 12 |
The infection advances to the next root via the root junction or perhaps from new infections. Smaller roots die while lesions are formed predominantly on larger roots. The fungus continues to colonize the root tissue and invades the xylem causing the plant to form tyloses. While the fungus invades the xylem, it does become systemic in the plant and, as such, is not a true vascular wilt pathogen, such as Fusarium oxysporum or Verticillium spp. Infection is favored by warm soil temperatures (25 to 35 °C). In climates where double cropping of melons occurs (two crops per year), disease typically is more severe in the late-summer or autumn-planted crop than the spring or winter-planted crop. Perithecia are formed on the roots most abundantly late in the season but may form throughout the life cycle. When mature, the perithecia rupture, discharging the ascospores into the soil (F, Figures 4, 5). Several hundred ascospores may form in a single perithecium (Figure 12) and a single mature melon plant root system is capable of producing 400,000 or more ascospores.
External stresses, both biotic and abiotic, may exacerbate disease symptoms and speed up wilting and vine collapse. One aspect that has been investigated in this regard is fruit load. Vine collapse usually occurs during fruit maturation and several studies have noted that removal of developing fruit from infected plants delays symptom expression and, in some cases, prevents wilting and death of the plant.
Above ground disease symptoms involve a gradual decline of the leaves, typically beginning with the crown leaves and progressing outward. By late in the season, many of the leaves are dead, exposing the developing fruit to intense solar radiation and heat. The combination of tyloses in the root tissue and the loss of most of the secondary and tertiary roots causes the vines to collapse quickly, often appearing as though they collapsed “overnight”. Entire fields of plants may collapse resulting in near total economic loss. This rapid collapse resulted in disease names such as 'sudden wilt' and 'sudden death'. Fruits on infected plants are of low quality due to reduced size and sugar content, as well as, sunburned and cracked rinds.
A PCR-based protocol using species-specific ITS primers has been developed for the early detection of M. cannonballus in root tissue. Similarly, a quantitative real-time PCR protocol has been developed, also based on the ITS1 region of the rDNA. This later technique has been used along with color image analysis to phenotypically score melon root responses to M. cannonballus.
Epidemiology
No aerial stage of the disease is known, and no asexual spore stage of the fungus has been observed. Thus, ascospores are considered the primary inoculum and can survive in the soil or plant debris until the next planting season or, in the absence of a host, may survive for many years in a dormant state. Mycelium from infected crop debris can serve as a source of inoculum, although it is not known how long mycelium survives in the soil. The disease is considered monocyclic.
Ascospores can build up to high numbers in fields cropped successively to melons; however, the minimum threshold level for disease development is not known. Severe disease has occurred in fields with as few as one to two ascospores per gram of dry soil and as high as 15 spores per gram of dry soil. In the few cases studied, ascospores appear uniformly distributed throughout the fields, with the highest concentration in the upper 10 inches (25 cm). There is some evidence that the fungus may colonize and reproduce on roots of other plants (monocots and dicots) without causing noticeable disease, providing another means of survival and reproduction. Additionally, ascospores have been found in soil from noncultivated land and in native desert soils in Texas and Arizona in the U.S. and in Brazil. Thus, M. cannonballus appears to be an indigenous soilborne fungus and the epidemiology of this disease may be more complex than we currently understand.
The spread of the pathogen can be by any method that displaces contaminated soil or crop debris e.g. cultivation, flooding, wind, erosion, etc. Airborne spread of the pathogen is not likely because of the large size of the ascospores and the lack of a known conidial stage. There is no evidence that the pathogen is seedborne or systemic in the plant. Colonization appears to be limited to the roots. Fruit and foliage are not infected directly, although they manifest symptoms related to root infection, water stress, and plant decline.
Disease Management
Control of MRR/VD has proven to be difficult. At present, there is no one method available that is both cost effective and long lasting that provides adequate control of the disease, although research using several different strategies is ongoing in several laboratories. Factors contributing to the difficulty in controlling MRR/VD include:
- intensive and successive cropping of melons, allowing for the buildup of inoculum,
- presence of numerous thick-walled ascospores that may reside in the soil for years and which are apparently tolerant to desiccation and chemicals,
- the general difficulty in applying and incorporating pesticides into the soil environment,
- the inherent buffering capacity of the soil and plant rhizosphere,
- the apparent wide host range of the fungus and its ability to colonize and reproduce on several monocot and dicot plants,
- cropping practices such as plastic mulch and buried drip irrigation that create a favorable soil environment for infection and survival of the fungus,
- cultural practices that result in poorly developed and shallow root systems, and
- lack of melon genotypes that are resistant or highly tolerant to infection and disease.
In spite of these obstacles, progress is being made in managing this disease. An integrated approach to the management of MRR/VD may be the best strategy. Integration increases the chance of developing effective management programs by combining partially effective methods, reducing the chances of negative side effects, and providing flexibility in adapting the control programs to different agricultural situations.
Chemical Soil Treatment. The most effective technique so far has been preplant soil fumigation with methyl bromide (Figure 13), chloropicrin, 1,3 dichloropropene, or metam sodium (Vapam™). However, the phase-out of methyl bromide and the increasing restrictions on fumigants, in general, make this a tenuous strategy. Other compounds have been applied experimentally via drip irrigation and have shown various degrees of control. Fluazinam, (Frowncide™ ISK, Japan) applied experimentally via drip irrigation during the season effectively controlled disease in Israel during the spring planting but was less effective in the late-summer crop; however, fluazinam is not labeled for use on cucurbits in the U.S. Fludioxinil (Cannonball™, Syngenta) is registered for control of M. cannonballus on melons and watermelons when applied via drip irrigation. A multi-phased strategy consisting of a preplant soil fumigation to reduce the resident inoculum in the soil, a postplant application of fungicide to inhibit root colonization during the season, and a postharvest cultivation of plants along with an immediate postharvest application of metam sodium (Vapam™) to prevent ascospore production has been developed for control of MRR/VD in California.
 Figure 13. |
Soil solarization. Studies in both the U.S. and Israel using conventional soil solarization were ineffective in controlling MRR/VD, presumably due to the ability of the fungus to grow at high temperatures. However, a modified method of solarization in open containerized plants and the combination of soil solarization with reduced rates of fumigation have shown good potential for control of MRR/VD, as well as other soilborne diseases in Israel.
Genetic resistance. Like most soilborne diseases, genetic resistance to MRR/VD is the method of choice for control; however, a good source of resistance to M. cannonballus has not yet been identified. Several laboratories are actively pursuing sources of resistance, and a few melon lines have been identified that exhibit tolerance to MRR/VD, including several accessions of C. melo subsp.agrestis and several Galia and Ananas-type melons. Thus far, no U.S. western-shipper type melons have shown any resistance or tolerance to M. cannonballus. Research has demonstrated the importance of a well-developed and vigorous root system for withstanding vine decline and root architecture is being evaluated as a phenotypic trait for tolerance and / or resistance. Root strucutre is believed to be a major factor in the grafting of susceptible melons onto rootstock of Cucurbita spp. and gourds for control of MRR/VD (see Grafting below).
Grafting. Other attempts at controlling MRR/VD include grafting susceptible melons (muskmelon and watermelon) onto Cucurbita spp. or Lagenaria spp. (bottle gourd) rootstock. This practice is used most commonly in Asia and the Mediterranean basin for the control of several soilborne diseases, primarily Fusarium wilt, but increasingly for management of MRR/VD. There is generally good rootstock-scion compatibility among many of the cucurbit species, and the large and extensive root systems of these winter squashes and gourds allow them to survive infection during the season. The use of these rootstocks should be approached with some caution, however as they are susceptible to infection by M. cannonballus and, therefore, could aid in the potential build-up of inoculum in the soil, albeit, they typically do not support extensive perithecia development on the roots.
Biological control. The use of hypovirulent isolates of M. cannonballus was investigated as a biological control for MRR/VD. Isolates infected with one or more double-stranded (ds) RNAs often result in cultures that are greatly reduced in virulence (hypovirulent) (Figure 14 ). These dsRNAs can be transmitted to normal, wild-type virulent isolates via hyphal anastomosis (fusion), rendering them hypovirulent. Subsequent "curing" of the infected isolates by extended growth at high temperature restores them to their wild-type pathogenicity. Thus far, transmissible hypovirulence has only been shown to have disease control potential in greenhouse experiments (Figure 15). Additionally, treatment of melon seeds with methyl jasmonate, a known inducer of pathogen defense mechanisms in plants, reduced the severity of MRR/VD under experimental conditions and offers an additional potential control strategy in the future.
 Figure 14 |
 Figure 15 |
Significance
Monosporascus root rot and vine decline is an emerging disease worldwide, and one that only recently has gained attention among plant pathologists. It is the primary pathogen of late-season vine declines of melons in most melon-growing areas of the world. The causal agent, Monosporascus cannonballus, was described as a genus et species novus by Pollack and Uecker in 1974 based on specimens obtained from necrotic muskmelon roots from Arizona. No pathogenicity trials were conducted at that time. The first confirmed report of pathogenicity was from Israel in 1983 where it was shown to the cause of a mature melon plant collapse, although the pathogen was identified as M. eutypoides. An earlier report from Japan indicated that this fungus was nonpathogenic. Pathogenicity of isolates from the United States was first reported in 1991 by Mertely et al. in Texas, and the disease was named Monosporascus root rot and vine decline (MRR/VD). By 1997 the disease had been reported from Texas, Arizona, and California in the US and from 13 other countries. It has since been reported in four additional countries bringing the total number of countries reporting MRR/VD in 2009 to 18. (Figure 16, Table 1). It is highly probable it will be detected in additional countries in the near future.
 Figure 16. |
TABLE 1. Countries that have reported either Monosporasucs cannonballus or M. eutypoides |
Country |
Year reported |
Reference |
USA (AZ) |
1974 |
Pollack, F.G. & Uecker, F.A. Mycology 66:346 |
USA (TX) |
1990 |
Mertely, J.C., et al. Plant Dis. 75:1133 |
USA (CA) |
1995 |
Bruton, B.D., et al. Plant Dis. 79:754 |
Libya |
1978 |
Hawksworth, D.L. & Ciccarone, A. Mycopathologia 66:147 |
India |
1978 |
Hawksworth, D.L. & Ciccarone, A. Mycopathologia 66:147 |
Japan |
1979 |
Watanabe, T. Trans. Mycol. Soc. Japan 20:312; |
|
1985 |
Uematsu, S., et al. Ann. Phytopathol Soc. Japan 51:272. |
Israel |
1983 |
Reuveni, R., et al., Trans. Br. Mycol. Soc. 80:354 |
Spain |
1990 |
Lobo-Ruano, M. Bol. San. Veg. Plagas 16:701 |
Tunisia |
1994 |
Martyn, R.D., et al. Plant Dis. 78:1220 |
Korea |
1994 |
Park, K.S., et al. Korean J. Plant Pathol. 10:175 |
Taiwan (R.O.C.) |
1995 |
Tsay, J-G & Tung, B-K. Plant Pathol. Bull.4:25 |
Mexico |
1996 |
Martyn, R.D., et al. Plant Dis. 80:1430 |
Saudi Arabia |
1997 |
Karlatti, R.S., et al. Plant Dis. 81:1215 |
Guatemala |
1997 |
Bruton, B.D. & Miller, M.E. Plant Dis. 81:694 |
Honduras |
1997 |
Bruton, B.D. & Miller, M.E. Plant Dis. 81:696 |
Italy |
1999 |
Gennari, S., et al. Infomatore Fitopatologica 49:38 |
Brazil |
2004 |
Sales, Jr., R., et al. Plant Dis. 88:84 |
Iran |
2008 |
Sarpeleh, A. Aust. Plant Dis. Notes 3:162 |
Iraq |
? |
|
Pakistan |
? |
|
Losses from MRR/VD can be quite extensive. The pathogen appears to be uniformly dispersed throughout fields causing widespread and uniform infection. Damage is done to the root system throughout the growing season and, typically, within a week or two of harvest, the vines wilt or collapse quickly and completely. This results in plants whose fruit are exposed to direct solar radiation and often crack and sunburn. In addition, since much of the sugar content is partitioned into the fruit in the last few weeks of growth, fruit from infected vines are low in sugar and of poor quality and size. In many cases, there can be total economic loss.
Monosporascus root rot and vine decline is an example of a vine decline disease of melon. Vine decline is a generic term used to describe a recalcitrant group of "diseases" with similar symptoms but different putative causal agents. General symptoms of vine declines include yellowing and death of the crown leaves and a gradual decline of the vine as the plant approaches maturity. A rapid collapse of the vine occurs late in the season, typically just before harvest. The primary effect of the infection is destruction of the roots that restricts water uptake and transport late in the season when the plant is experiencing a high water demand due to increased stomatal transpiration and water transport to the fruit resultin in vines that collapse almost "overnight." Vine declines have become increasingly more prominent in the last 25 years.
A common question is: "Why has this disease become so prominent in the last decade?" There is no definitive answer to this, but there are several factors that probably have contributed to it. First, it probably did not appear as a new disease in the 1980s, but rather was already present in a number of places, but either was not identified or was misidentified. Once it was reported as a pathogen of melons, other laboratories perhaps were inclined to look more carefully. Additionally, there are several cultural and cropping conditions in melons that have changed radically in the last 25 years that are coincident with the increase in vine decline diseases: a) the virtually complete shift from open-pollinated melons and watermelons to hybrids, resulting in plants with an earlier and more concentrated fruit set; b) the shift from direct seeding to the use of seedling transplants; and c) the shift from growing plants on bare ground with furrow or overhead irrigation to the use of plastic mulch and drip irrigation. These latter two practices result in plants with poorly developed and shallow root systems that tend to grow parallel beneath the plastic instead of penetrating deep into the soil profile. And lastly, with higher inputs and production costs, many growers have shortened their rotation schemes and are reusing fields sooner or, in some cases, continually cropping melons for two or more successive seasons. This practice significantly adds to the inoculum build-up in the soil.
All of these factors may affect the overall condition of the plant and predispose it to infection. Another factor to consider is that over the last 25 years, more and more non-crop land has been cleared and planted to watermelons and melons. There is some evidence that M. cannonballus is a native or indigenous pathogen. The planting of highly susceptible plants, such as melons, may have selected for or baited the fungus and allowed its rapid increase. Since new melon varieties and cultural practices often are quickly adopted by growers around the world, it is possible that the selection for the pathogen also occurred quickly. While there are no hard data to support this hypothesis, it warrants some consideration and testing.
M. cannonballus is a devastating pathogen of melons wherever it occurs, but it cannot explain or account for all cases of vine declines and collapses seen around the world. In cases where M. cannonballus has not been found, other organisms have been implicated in the disease. Most of these are fungi, but viruses, bacteria and even herbicides also have been implicated. In other cases, no definitive causal agents have been described. A significant contributor to the vine decline syndrome is a poorly developed root system. Transplanted seedlings grown in traditional small cells typically do not develop strong taproots (Figure 17). As the taproot elongates and reaches the bottom of the transplant cell, it curls around the edges, resulting in a taproot that either quits growing or continues in a circular, twisted configuration along the sides of the transplant cell. When transplanted into the field, the taproot does not recover and grow down into the soil profile, but rather produces a plant with a proliferation of lateral roots growing horizontally along the transplant bed following the water stream but having no significant taproot (Figure 17). By the end of the season, when water demand is high, the compromised root system labors to keep up. At this stage, it doesn’t take much additional stress to cause collapse. Cultural methods that improve root development can help alleviate many of the vine decline symptoms seen around the world. Lengthening the transplant container, such as with the use of Cone-tainers™, (Figure 18), typically used for pine seedlings and other trees, will allow the taproot to develop more fully (Figure 19). Additionally, the use of improved irrigation techniques such as dual drip tapes buried approximately 15 to 20 cm below the soil surface, on either side of the plants places the water deeper in the soil and on the outside of the beds, forcing the roots to explore more of the soil profile, ultimately producing a more vigorous root system that can better support the demand of the vines and fruit late in the season and withstand minor stresses (Figure 20). Treatment of soilless potting mixtures with one or more mycorrhizal fungi prior to seeding also may improve root development. Direct seeded plants, however, still typically produce the best roots and vines.
 Figure 17. |
 Figure 18 |
 Figure 19 |
 Figure 20. |
Selected References
Batten, J. S., K-B. Scholthof, B.R. Lovic, M.E. Miller, and R.D. Martyn. 2000. Potential for biocontrol of Monosporascus root rot / vine decline under greenhouse conditions using hypovirulent isolates of Monosporascus cannonballus. European Journal of Plant Pathology 106:639-649.
Cohen, R., S. Pivonia, Y. Burger, M. Edelstein, A. Gamliel, and J. Katan, J. 2000. Toward integrated management of Monosporascus wilt of melons in Israel.Plant Dis. 84:496-505.
Egel, D.S., R. Martyn, and C. Gunter. 2008. Planting method, plastic mulch, and fumigation influence growth, yield, and root structure of watermelon. HortScience 43:1410-1414.
Miller, M.E., R.D. Martyn, and B.D. Bruton. 2000. Muskmelon growth and yield in response to fumigation. Acta Horticulturae 510:179-185.
Martyn, R.D. 2007. Late-season vine declines of melons: Pathological, cultural or both? Acta Horticulturae 731:345-356.
Martyn, R. D. and M. E. Miller. 1996. Monosporascus root rot/vine decline: An emerging disease of melons worldwide. Plant Disease 80:716-725.
Martyn, R. D. and M. E. Miller. 1996. Monosporascus root rot/vine decline of muskmelon and watermelon. Pages 18-19.in: T. A. Zitter, D. A. Hopkins, and C. E. Thomas (eds.), Compendium of Cucurbit Diseases, APS Press, St. Paul, MN.
Dias, R., B. Picó, A. Espinos, and F. Nuez. 2004. Resistance to melon vine decline derived from Cucumis melo ssp.agrestis: Genetic analysis of root structure and root response. Plant Breeding 123:66-72.
Pollack, F. G., and F. A. Uecker. 1974.Monosporascus cannonballus, an unusual Ascomycete in cantaloupe roots. Mycologia 66:346-349.
Radewald, K.C., D.M. Ferrin, and M.E. Stanghellini. 2004. Sanitation practices that inhibit reproduction of Monosporascus cannonballus in melon roots left in the field after crop termination. Plant Pathology 53:660-668.
Stanghellini, M. E., D.H. Kim, and S.L. Rasmussen. 1996. Ascospores of Monosporascus cannonballus: Germination and distribution in cultivated and desert soils in Arizona. Phytopathology 86:509-514.
Waugh, M.M., D.M. Ferrin, and M.E. Stanghellini. 2005. Colonization of cantaloupe roots by Monosporascus cannonballus. Mycological Research 109:1297-1301.