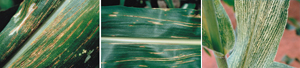 Figure 1 |
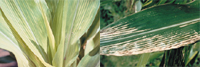 Figure 2 |
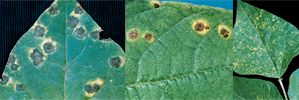 Figure 3 |
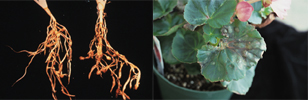 Figure 4 |
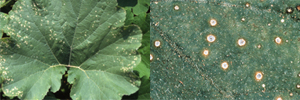 Figure 5 |
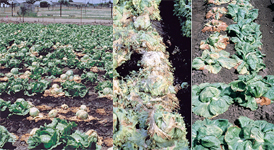 Figure 6 |
Fungicides, herbicides and insecticides are all pesticides used in plant protection. A fungicide is a specific type of pesticide that controls fungal disease by specifically inhibiting or killing the fungus causing the disease. Not all diseases caused by fungi can be adequately controlled by fungicides. These include the vascular diseases Fusarium and Verticillium wilt (Figure 7). Diseases caused by other types of organisms, disorders caused by abiotic factors, and insect damage are not controlled by fungicides. Thus it is essential to first determine the cause of symptoms before applying a fungicide.
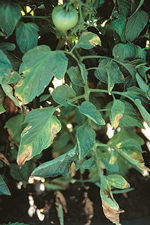 Figure 7 |
Why are Fungicides Needed?
Diseases are a common occurrence on plants, often having a significant economic impact on yield and quality, thus managing diseases is an essential component of production for most crops. Broadly, there are three main reasons fungicides are used: (a) To control a disease during the establishment and development of a crop. (b) To increase productivity of a crop and to reduce blemishes. Diseased food crops may produce less because their leaves, which are needed for photosynthesis, are affected by the disease (Figures 8 - 11). Blemishes can affect the edible part of the crop (Figures 6 and 14) or, in the case of ornamentals, their attractiveness (Figures 12 - 13), which both can affect the market value of the crop. (c) To improve the storage life and quality of harvested plants and produce. Some of the greatest disease losses occur post-harvest (Figures 14 and 15). Fungi often spoil (render unusable) stored fruits, vegetables, tubers, and seeds. A few which infect grains produce toxins (mycotoxins) capable of causing severe illness or even death in humans and animals when consumed. Fungicides have been used to reduce mycotoxin contamination in wheat affected by Fusarium head blight, but most fungicides developed so far have not been sufficiently effective to be useful for managing mycotoxins associated with other diseases.
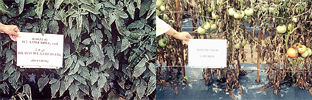 Figure 8 |
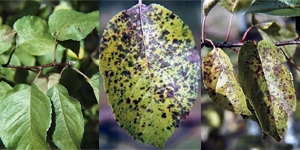 Figure 9 |
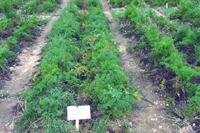 Figure 10 |
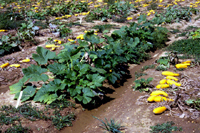 Figure 11 |
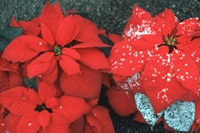 Figure 12 |
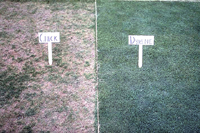 Figure 13 |
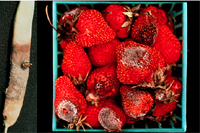 Figure 14 |
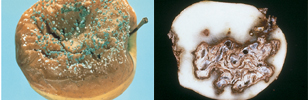 Figure 15 |
Role of Fungicides in Disease Management
Plant diseases are best managed by integrating a number of control practices that may include: crop rotation, selection of disease-tolerant or disease-resistant crop cultivars (cultivars genetically less susceptible than other cultivars), time of planting, level of fertilization, micro-climate modification, sanitation, and application of fungicides. Fungicides are often a vital part of disease management as (a) they control many diseases satisfactorily, (b) cultural practices often do not provide adequate disease control, (c) resistant cultivars are not available or not accepted in the marketplace for many diseases, and (d) certain high value crops have an extremely low tolerance for disease symptoms.
In contrast with most human medicines, most fungicides need to be applied before disease occurs or at the first appearance of symptoms to be effective. Unlike with many diseases of humans and animals, applying fungicides cannot heal symptoms already present, even if the pathogen is killed. This is because plants grow and develop differently than animals. Fungicides typically only protect new uninfected growth from disease. Few fungicides are effective against pathogens after they have infected a plant. Those that do have “curative” properties, which means they are active against pathogens that have already infected the plant, have limited ability to do so, often only being active on a pathogen within a few days of infection.
Many fungicides have targeted activity that imparts high efficacy against specific pathogens, which means low potential for toxicity to humans and other organisms, but also results in a high risk of pathogens developing resistance to the fungicide. A resistant pathogen is less sensitive to the action of the fungicide, which results in the fungicide being less effective or even ineffective. Fungicides that are designed to target specific enzymes or proteins made by fungi do not damage plant tissue, thus they can penetrate and move inside leaves enabling curative properties and increasing the amount of plant tissue protected to more than just where fungicide was deposit when applied. Since the mode of action of these fungicides is so specific, small genetic changes in fungi can overcome the effectiveness of these fungicides and pathogen populations can become resistant to future applications. Disease management strategies that rely heavily upon curative application of fungicides often lead to more resistance problems due to (a) the large size of the pathogen population when the application is made from which resistant individuals are being selected and (b) the difficultly in eradicating a pathogen entirely from inside the plant. Fungicide resistance is covered in more detail in a separate section.
Growers often use disease forecasting systems or action thresholds, when these are available, to ensure fungicides are applied when needed and to avoid the expense and possible environmental impact of unnecessary applications. Forecasting systems have been developed for a number of diseases based on an understanding of the environmental conditions favorable for their development. Typically these are based on temperature and relative humidity or leaf wetness in the area where the crop is grown. Threshold-based fungicide programs involve routinely scouting the crop for symptoms, then applying fungicides when the amount of symptoms reaches a critical level beyond which the disease cannot be controlled adequately. An example of a critical level is one disease spot per five leaves examined. Knowledge of the disease cycle of the pathogen is important when developing and using forecasting systems and thresholds. Important aspects of the disease cycle include whether the disease is monocyclic (one generation per year) or polycyclic (multiple generations) and latent period (time between infection and production of new inoculum).
Economics often influence the choice of fungicide and application timing. Expensive fungicides and numerous applications are used on valuable plantings that might incur substantial economic loss in the absence of treatment, such as fruit trees and golf courses. Recognizing that with some diseases crop yield is not impacted when severity is low, an economic threshold is used to determine when fungicide treatment is needed. The crop tolerance level, or damage threshold, can vary depending upon the stage of the crop development when attacked, crop management practices, location and climatic conditions.
Fungicides are applied as dust, granules, gas, and, most commonly, liquid. They are applied to:
- (a) Seed, bulbs, roots of transplants, and other propagative organs. These treatments are usually done by the seed company. Some treatments need to be done by the grower on-site at the time of planting. The goal is to kill pathogens that are on the planting material or to protect the young plant from pathogens in the soil.
- (b) Soil either in-furrow at planting, after planting as a soil drench (including through drip irrigation), or as a directed spray around the base of the plant.
- (c) Foliage and other aboveground parts of plants by means of a sprayer.
- (d) Inside of trees via trunk injection.(f) Harvested produce, as a dip or spray in the packinghouse.
Fungicides are used as a formulated product consisting of an active ingredient plus inert ingredients that improve the performance of the product. Fungicides are typically mixed with water then applied by spraying. Application equipment ranges from small hand-held and back-pack sprayers to large spray units carried by tractors or aircraft (Figures 16-22). A few fungicides are applied as dusts. Fungicides can also be applied in greenhouses as smoke, mist, fog or aerosol. Coverage of all parts of the plant susceptible to the disease is critical because very few fungicides can move adequately throughout a plant. Advancements are continually being made to nozzles and sprayers to improve coverage (Figures 17 and 19).
For many diseases, effective control necessitates multiple applications of fungicides, sometimes as frequently as every 5 days. Repeated applications are needed to protect new growth and to replace fungicide lost from the plant by chemical decomposition, UV-light degradation, and erosion by wind and water.
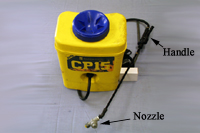 Figure 16 |
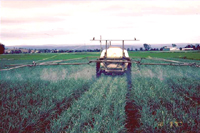 Figure 17 |
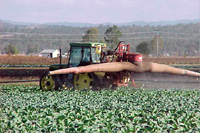 Figure 18 |
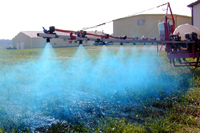 Figure 19 |
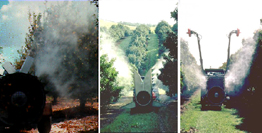 Figure 20 |
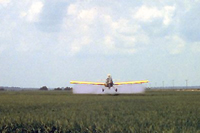 Figure 21 |
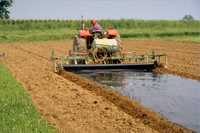 Figure 22 |
Types of Fungicides and Definitions
Fungicides are categorized in several ways based on different characteristics. The most common characteristics used and the categories are described below. Table 1 (Adobe Acrobat PDF) is a list of selected fungicides currently registered in the United States that represent the major fungicide groups and chemistry within these groups.
- Mobility in a plant: contacts or systemics: Contact fungicides (also called protectants) remain on the surface of plants. Many contacts are potentially phytotoxic (toxic to plants) and can damage the plant if absorbed. Systemics (also called penetrants and mobile fungicides) are absorbed into plants. Most systemics move very short distances from the site of application, such as across a leaf blade from one surface to the other (local systemic or translaminar). Some fungicides are weakly systemic and can move further from the application site than local systemics, reaching all parts of the leaf the fungicide is deposited on. A few systemics move more extensively because they are mobile in xylem tissue. When applied to the root zone, these are absorbed by roots and then move upward through the plant with the transpiration stream (xylem-mobile systemic). Xylem-mobile systemics applied to leaves move throughout the leaf where deposited, but cannot be redistributed out of that leaf; however, any material deposited on stems can move upwards into leaves. Phloem-mobile systemics (also known as “true” or amphimobile systemics) have bi-directional mobility, some material moves in phloem out of the leaf where deposited upwards to other leaves and downwards to roots. Systemics cannot move again after translocation.
Animated examples of fungicide mobility:
Contact fungicide - applied to foliage.
Local systemic or translaminar- applied to foliage.
Xylem mobile systemic - applied to soil.
Xylem mobile systemic - applied to foliage.
Amphimobile systemic - applied to foliage.
- Role in protection: preventive or curative. Contacts are products suited for preventive (prophylactic) use as they work by contact action on the surface of the plant to which they have been applied. Repeated applications are needed to protect new growth of the plant and to replace material that has been washed off by rain or irrigation, or degraded by environmental factors such as sunlight. Sometimes contacts are referred to as “residual” products because the deposited fungicide remains on the plant surface, occasionally as a visible residue, for several days. Due to their ability to penetrate plants, some systemics possess both preventive and curative (eradicant or kick-back) activity, thus affecting the pathogen after infection.
- Breadth of activity: single-site or multi-site. Single-site fungicides are active against only one point in one metabolic pathway in a pathogen or against a single critical enzyme or protein needed by the fungus. Since single-site fungicides are highly specific in their toxicity, having little effect on most organisms, they can safely be absorbed into plants, thus these fungicides tend to have systemic properties. As a result of this specific activity, fungi are more likely to become resistant to the fungicide because a single mutation in the pathogen usually allows it to overcome the action of the fungicide, such as by preventing it from binding to the active site in the fungus.
Typically, older contact fungicides have multi-site activity and thus usually affect many fungi in different classes. Through the development of in vivo screens, and due to the increase in the stringency and number of regulatory tests required to register a new active ingredient, fungicide manufacturers have found it easier to develop single-site systemics recently. As a result, fungicide resistance has become a more important concern in disease management.
- Mode of action. Fungicides kill fungi by damaging their cell membranes, inactivating critical enzymes or proteins, or by interfering with key processes such as energy production or respiration. Others impact specific metabolic pathways such as the production of sterols or chitin. For example, phenylamide fungicides bind to and inhibit the function of RNA polymerase in oomycetes, while the benzimidazole fungicides inhibit the formation of beta tubulin polymers used by cells during nuclear division.
Some recently developed products are unique in that they do not directly affect the pathogen itself. Many of these elicit a response from the host plant known as "systemic acquired resistance" (SAR). These SAR inducers basically mimic chemical signals in plants that activate plant defense mechanisms such as the production of thicker cell walls and anti-fungal proteins. The utility of SAR inducers, however, has been limited so far since many pathogens are capable of over-powering such defenses.
Knowledge of exactly how a fungicide affects a fungus is helpful for selecting products. First, mode of action determines which fungi will be affected by a fungicide and thus which diseases can be controlled by using the fungicide. Second, fungicides with different modes of action are needed in a disease management program to delay fungicide resistance development.
Biochemical mode of action is the primary basis used to classify fungicides into chemical groups. A list of fungicides organized by chemical group is maintained by the Fungicide Resistance Action Committee (FRAC) (http://www.frac.info/frac/index.htm) and is in Appendix II of a US Environmental Protection Agency (EPA) document (www.epa.gov/opppmsd1/PR_Notices/pr2001-5.pdf). Fungicides in Table 1 (Adobe Acrobat PDF) are listed according to the FRAC/EPA classification scheme plus an additional class for biofungicides. Basic chemical information for fungicides is at the PAN Pesticide Database (www.pesticideinfo.org). All fungicides within a chemical group share a common mode of action and resistance mechanism, even though their chemical structures may be different. Two exceptions in the FRAC/EPA classification scheme are the “multi-site activity” fungicide class and the “unknown” class of fungicides with unknown modes of action.
- Breadth of activity: narrow-spectrum or broad-spectrum. Narrow-spectrum fungicides are effective against only a few usually closely related pathogens. These usually have single-site activity and are often systemic. Broad-spectrum fungicides can often control a wide range of unrelated pathogens. These usually are contacts with multi-site activity, but some have single-site activity. Several fungicides have activity that places them on a continuum between narrow- and broad-spectrum fungicides.
- Type of chemical: inorganic or organic. Fungicides also can be classified based upon their chemical composition. Chemically, organic molecules are those that contain carbon atoms in their structure whereas inorganic molecules do not. Many of the first fungicides developed were inorganic compounds based on sulfur or metal ions such as copper, tin, cadmium and mercury that are toxic to fungi. Copper and sulfur are still widely used. Most other fungicides used today are organic compounds and thus contain carbon. The term "organic" as used here is based on chemistry terminology and differs from "organic" used to describe a system of agriculture that strives to be holistic and to enhance agroecosystem health.
Table 1 (Adobe Acrobat PDF) provides a list of fungicides and their characteristics that are representative of the major chemical groups registered in the USA.
- Additional terms.
- Active ingredient (a.i.) is the active component of a pesticide, and is that moiety which is patented, synthesized, and registered by the manufacturer.
- Biofungicide is a naturally based microbial or biochemical product. There are three types of biopesticides. (a) Microbial biopesticides have an active ingredient that is a biological control agent (organism capable of attacking or competing with a pathogen or pest). (b) Plant biopesticides or plant-incorporated protectants are defined by the EPA as "pesticidal substances that plants produce from genetic material that has been added to the plant". (c) Biochemical biopesticides contain naturally-occurring substances. Some biochemicals may also be products of fermentation. Biochemicals can directly affect disease-causing organisms or may stimulate SAR. Biopesticides generally are narrow-spectrum, have low toxicity, decompose quickly, and thus are considered to have low potential for negative impact on the environment (www.epa.gov/pesticides/biopesticides/). Many biofungicide products are being approved for organic crop production (www.omri.org). While many have low toxicity, biopesticides are not necessarily safer than pesticides containing synthetic ingredients.
- Chemical group or class is the name given to a group of chemicals that share a common biochemical mode of action and may or may not have similar chemical structure.
- Chemical name is denoted by a nomenclature system designed by the International Union of Pure and Applied Chemistry (IUPAC); this set of rules is used to name organic, carbon-based compounds.
- Common name is the name proposed by the basic manufacturer for the active ingredient. This name is ratified by a committee either at IUPAC or the ACS (American Chemical Society).
- Reduced risk is a special classification approved by the EPA for specific uses of pesticides that have low risk to human health, low toxicity to non-target organisms, low potential to contaminate water or other environmental resources, and/or that broaden the adoption and effectiveness of integrated pest management strategies. Registration is expedited for uses designated as reduced risk.
- Formulation is the pesticide product. It is a prepared mix of active ingredient plus ingredients that improve pesticidal performance, such as carriers, solvents, wetting agents, spreaders, and stickers. Types of formulations include wettable powders, dry flowables, emulsifiable concentrates, and granules.
- Trade name/Trademark is the patented name under which a product is sold to the end user.
Fungicide Resistance
Fungicide resistance is a stable, heritable trait that results in a reduction in sensitivity to a fungicide by an individual fungus. This ability is obtained through evolutionary processes. Fungicides with single-site mode of action are at relatively high risk for resistance development compared to those with multi-side mode of action. Most fungicides being developed today have a single-site mode of action because this is associated with lower potential for negative impact on the environment, including non-target organisms.
When fungicide resistance results from modification of a single major gene, pathogen subpopulations are either sensitive or highly resistant to the pesticide. Resistance in this case is seen as complete loss of disease control that cannot be regained by using higher rates or more frequent fungicide applications. This type of resistance is commonly referred to as “qualitative resistance”.
When fungicide resistance results from modification of several interacting genes, pathogen isolates exhibit a range in sensitivity to the fungicide depending on the number of gene changes. Variation in sensitivity within the population is continuous. Resistance in this case is seen as an erosion of disease control that can be regained by using higher rates or more frequent applications. Long-term selection for resistance in the pathogen by repeated applications may eventually result in the highest labeled rates and/or shortest application intervals not being able to adequately control the disease. This type of fungicide resistance is commonly referred to as “quantitative resistance”. Comments about resistance risk of fungicides are included in Table 1 (Adobe Acrobat PDF) and in a table of fungicides at the FRAC web site (http://www.frac.info/home/).
Fungal isolates that are resistant to one fungicide are often also resistant to other closely-related fungicides, even when they have not been exposed to these other fungicides, because these fungicides all have similar mode of action. This is called cross resistance. Fungicides with the same Group Code are likely to exhibit cross resistance. Occasionally negative cross resistance occurs between unrelated fungicides because the genetic change that confers resistance to one fungicide makes the resistant isolate more sensitive to another fungicide.
Managing fungicide resistance is critically important to extend the period of time that an at-risk fungicide is effective. The primary goal of resistance management is to delay its development rather than to manage resistant fungal strains after they have been selected. Therefore, resistance management programs need to be implemented when at-risk fungicides first become available for commercial use. The objective of resistance management is to minimize use of the at-risk fungicide without sacrificing disease control. This is accomplished by using the at-risk fungicide with other fungicides and with non-chemical control measures, such as disease resistant cultivars, in an integrated disease management program.
It is critical to use an effective disease management program to delay the build-up of resistant strains. At-risk fungicides should be used at the manufacturer’s recommended rate (full rate) and application interval. Using full rates is expected to minimize selection of strains with intermediate fungicide sensitivity when resistance involves several genes (quantitative resistance). At-risk fungicides should be used in alternation with other at-risk fungicides with different modes of action or different chemical groups, and they should be combined or alternated with fungicides that have a low resistance risk.
When one crop could serve as a source of inoculum for a subsequent crop, the alternation scheme among at-risk fungicides should be continued between successive crops such that the first at-risk fungicide applied to a crop belongs to a different cross-resistance group than the last at-risk fungicide applied to the previous crop. Some at-risk fungicides are formulated as premix products with other fungicides to manage resistance. At-risk fungicides should be used only when needed most. The most critical time to use them for resistance management is early in an epidemic when the pathogen population is small. Multi-site contact fungicides should be used alone late in the growing season, where they have been shown to provide sufficient disease control to protect yield. Another important component of resistance management is assessing disease control and reporting any loss of efficacy potentially due to resistance.
To promote resistance management, companies registering fungicides are voluntarily putting on the labels guidelines developed recently by EPA through a joint effort with the Canadian Pest Management Regulatory Agency (PMRA) under the North American Free Trade Agreement (NAFTA). These are described in Pesticide Registration (PR) Notice 2001-5 (www.epa.gov/opppmsd1/PR_Notices/pr2001-5.pdf). Group codes for designating chemical groups were developed as part of these guidelines (see Table 1 (Adobe Acrobat PDF)).
The two major laws governing fungicides and other pesticides in the United States are the Federal Insecticide, Fungicide, and Rodenticide Act (FIFRA), and Federal Food, Drug, and Cosmetic Act (FFDCA).
FIFRA was passed by Congress in 1947. Primary responsibility for its enforcement was initially with the United States Department of Agriculture (USDA), then transferred to the EPA in 1970. The Office of Pesticide Programs of EPA is chiefly responsible for regulating pesticides today. All pesticides must be registered before they can be sold and used in the U.S. unless their active and inert ingredients are deemed sufficient low risk to not require FIFRA regulation. To obtain registration, manufacturers of a pesticide must demonstrate that it does not have the potential to cause an adverse impact on the environment or non-target organisms, including people. This requires conducting several defined toxicology tests and investigating environmental fate. Additionally, the EPA must ensure that no endangered or threatened species or their habitat are harmed through use of registered pesticides. This assures compliance with the Endangered Species Act (ESA) of 1973 which prohibits any action that can adversely affect these species. In addition to federal registration with EPA, all pesticides must be registered with appropriate agencies in each state before they can be used.
FFDCA regulates the establishment of pesticide tolerances, which are the maximum permissible level of pesticide residues allowed in or on commodities for human food and animal feed. Manufacturers must include residue data in their registration materials. The Delaney Clause to FFDCA prohibited the presence in food of additives, including pesticides, considered carcinogenic. While well-intended, implementing this amendment became difficult as technology improvements enabled detection of additives at extremely low concentrations that were well below the dose necessary to cause cancer. Paradoxically, alternative pesticides could be allowed although they posed higher risks, if these were non-cancer risks. The Food Quality Protection Act (FQPA) passed in 1996 replaced the Delaney Clause with a new health-based standard for evaluating food-use pesticides that includes a ‘reasonable certainty of no harm’ provision. Under the new standard, EPA establishes tolerances by considering (a) aggregate exposure to a pesticide from food as well as residential and other non-food uses, (b) cumulative effects to human health from other pesticides with a common mode of toxicity, (c) potential of increased sensitivity of infants and children as compared to adults, and (d) effect of the pesticide on estrogen and the endocrine system. EPA is reevaluating all existing pesticide tolerances under FQPA. As a consequence of FQPA and stricter EPA standards for pesticide registration, some older pesticides are not being re-registered and it is more difficult to register new products.
A pesticide label is a legal document. Therefore it is against federal law to apply a pesticide in a manner other than that described on the label, such as using a higher rate or shorter application interval. Federal law requires specific information be included (pep.wsu.edu/factsheet/understanding.htm) (http://www.epa.gov/grtlakes/). Labels for fungicides registered in the USA are accessible on-line (www.cdms.net/manuf/manuf.asp)(www.epa.gov/pesticides/pestlabels). Figure 23 is a fictitious example fungicide label with the type of information found in most labels.
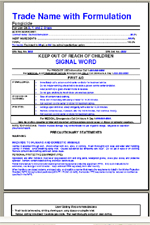 Figure 23 |
Pesticide applicators are affected by additional regulations as well, including the Worker Protection Standard (WPS). Some pesticides are considered restricted and consequently can only be applied by certified applicators who have passed an exam demonstrating an understanding of pesticides and safety (www.epa.gov/pesticides/health/worker.htm).
Additional information on pesticide regulations is available on-line (www.epa.gov/pesticides/regulating/index.htm). Information on potential hazards associated with a pesticide and directions for safe use are provided on the label and in its Material Safety Data Sheet (MSDS). An MSDS is required for all chemicals considered hazardous as defined by the U.S. Government's Occupational Safety and Health Administration (OSHA). MSDSs include information on physical data (melting point, boiling point, flash point etc.), toxicity, health effects, first aid, reactivity, storage, disposal, protective equipment, and spill/leak procedures (www.ilpi.com/msds/faq/parta.html#whatis).
- http://www.frac.info/home/
Select "Publications" in list on left. Under ‘FRAC Fungicide Lists’ at this site there are 2 lists: FRAC Fungicide List 1 contains fungicides arranged by FRAC Code and FRAC Fungicide List 2 contains fungicides sorted by mode of action. There are also two useful monographs on managing fungicide resistance and assessing resistance risk.
- nepis.epa.gov/Exe/ZyPDF.cgi/200000PL.PDF?Dockey=200000PL.PDF
This pdf file contains a pesticide classification system based on mode of action (target site) and voluntary pesticide resistance management labeling guidelines. It was prepared for those responsible for registration of pesticide products by the EPA Office of Prevention, Pesticides and Toxic Substances.
- www.pesticideinfo.org
The PAN Pesticide Database includes basic chemical information, toxicity characteristics, and regulatory status for numerous pesticides.
- www.epa.gov/pesticides/biopesticides/
Information about biopesticides and products available for use in the U.S. are at this website.
- www.omri.org
The Organic Materials Review Institute maintains lists of products submitted to them for review that they have determined to be acceptability for organic crop production in the U.S. under the National Organic Program.
- pep.wsu.edu/wp-content/uploads/sites/7/2015/05/learningaboutlabels.pdf
Information that must appear on a pesticide label by law is described at this site within the section where it is usually found on labels.
- iaspub.epa.gov/apex/pesticides/f?p=PPLS:1
The Pesticide Product Label System is a searchable collection of original labels for each pesticide approved by the US Office of Pesticide Programs, amended versions subsequently approved, and any associated correspondence about the terms of registration.
- extension.psu.edu/pests/pesticide-education/applicators/fact-sheets/pesticide-safety/toxicity-of-pesticides
A table with EPA’s Toxicity Rating Scale and corresponding signal word required on labels is at this site about the toxicity of pesticides.
- www.cdms.net/Label-Database
Labels and MSDSs for fungicides registered in the U.S. can be obtained by entering the product name in a search or by examining lists of products by manufacturer.
- www.epa.gov/pesticide-worker-safety/agricultural-worker-protection-standard-wps
Safety information and training materials are at this site for workers who will be handling pesticides or just entering areas where they were applied. This is part of EPA's Worker Protection Standard (WPS) for protecting agricultural workers from the effects of exposure to pesticides.
- www.epa.gov/pesticides
This site covers U.S. pesticide regulations and registration. It also has information about pesticides and their ingredients, tolerances, protecting health and the environment, assessing risk, pesticide reevaluation process, plus pesticide news articles.
- www.ilpi.com/msds/faq/parta.html#whatis
Material Safety Data Sheet (MSDS) information at this site includes their required elements and where to obtain them. Tutorials are available to learn how to read MSDS’s.
Selected Reading and References
- Carisse, O. 2010. Fungicides. InTech. http://www.intechopen.com/books/fungicides
- Fry, W. E. 1982. Principles of Plant Disease Management. Academic Press. New York, NY.
- Green, M. B. and D.A. Spilker. 1986. Fungicide Chemistry: Advances and Practical Applications (ACS Symposium Series, 304). American Chemical Society. Oxford University Press.
- Hutson, D. and Miyamoto, J. 1999. Fungicidal Activity: Chemical and Biological Approaches to Plant Protection. John Wiley & Sons. New York, NY.
- Koller, W. 1992. Target Sites of Fungicide Action. CRC Press. Baca Raton, FL.
- Lyr, H., Russell, P.E., and Sisler, H.D. 1996. Modern Fungicides and Antifungal Compounds. Intercept Limited. Andover, United Kingdom.
- Nene , Y. L. and Thapliyal, P. N. 1993. Fungicides in Plant Disease Control. Science Publishers, Inc. India.
- Oliver, R. P. and Hewitt, H. G. 2014. Fungicides in Crop Protection. 2nd edition. CABI Publishing, CAB International.
- Page, B. G. and Thomson, W.T. 2003. The 2003 Newly Revised Insecticide, Herbicide, Fungicide Quick Guide. Kovak Books. Bakersfield, CA.
- Smith, I.M. 1986. Fungicides for Crop Protection: 100 Years of Progress. International Specialized Book Services. Portland, OR.
Acknowledgements
I thank V. Morton for providing input throughout the preparation of this paper. I also thank M. Braverman, S. Broscious, H. Chen, J. Huether, R. Kaiser, S. Matten, M. Mahoney, and N. Ragsdale for reviewing drafts of this work and M. Daughtrey, G. Geitz, J. Hartman, S. A. Johnston, D. Rosenberger, P. Shoemaker, and P. Vincelli for providing figures.