Lambert, K. and S. Bekal. 2002. Introduction to Plant-Parasitic Nematodes.
The Plant Health Instructor. DOI: 10.1094/PHI-I-2002-1218-01
Revised 2009 by the Education Center Editorial Board
Kris Lambert and Sadia Bekal
University of Illinois, Department of Crop Sciences, Urbana, IL
The History of Nematodes
Members of the phylum Nematoda (round worms) have been in existence for an estimated one billion years, making them one of the most ancient and diverse types of animals on earth (Wang et al. 1999). They are thought to have evolved from simple animals some 400 million years before the "Cambrian explosion" of invertebrates able to be fossilized (Poinar 1983). The two nematode classes, the Chromadorea and Enoplea, have diverged so long ago, over 550 million years, that it is difficult to accurately know the age of the two lineages of the phylum (Figure 1).
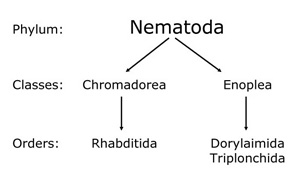 |
Figure 1. |
Nematodes are multicellular animals in the group Ecdysozoa, or animals that can shed their cuticle. Also included in this group with nematodes are insects, arachnids and crustaceans. In contrast to some of their relative invertebrates, nematodes are soft-bodied. Thus, very few nematodes have been fossilized (22 species from 11 genera) and exactly what ancestral nematodes looked like remains unknown. While we do not know the morphology of the first nematodes, it is probable that they were microbial feeders in the primordial oceans. The oldest known fossil nematodes are only 120-135 million years old; by then nematodes had diversified to feed on microbes, animals and plants (Poinar et al. 1994, Manum et al. 1994). The oldest fossil nematodes are found in amber and are commonly associated with insects. This is probably due to the fact that tree sap, which fossilizes to make amber, captures and preserves insects and their associated nematodes much more easily than an animal- or a nematode-infested portion of a plant. Much of what we know about the evolution of nematodes is inferred from the comparative anatomy of existing nematodes, trophic habits, and by the comparison of nematode DNA sequences (Thomas et al. 1997, Powers et al. 1993). Based upon molecular phylogenic analyses, it appears that nematodes have evolved their ability to parasitize animals and plants several times during their evolution (Blaxter et al. 1998). One point is clear; nematodes have evolved to fill almost every conceivable niche on earth that contains some amount of water. Nematodes are extremely abundant and diverse animals; only insects exceed their diversity. Most nematodes are free-living and feed on bacteria, fungi, protozoans and other nematode (40% of the described species); many are parasites of animals (invertebrates and vertebrates (44% of the described species) and plants (15% of the described species) .
Nematodes were noted early in human history because some serious human diseases are caused by relatively large vertebrate-parasitic nematodes. Some of these nematodes were first described in the ancient Chinese scientific literature as early as 2700 B.C. (Maggenti 1981). Since plant parasitic nematodes often are small and subterranean, there are not many ancient references to phytoparasitic nematodes. One interesting observation suggests that phytoparasitic nematodes were known in antiquity (235 B.C.) because the ancient Chinese symbol for a soybean root-infesting organism resembles in shape an adult female soybean cyst nematode (Noel, 1992). The first described plant parasitic nematodes were discovered in wheat seeds by Needham (1743). Not until the identification of root-knot nematodes on cucumber by Berkeley (1855) and cyst nematodes causing “beet-tired” disease on sugar beets by Schacht (1859), did plant nematology begin to emerge as an important scientific discipline. Nathan A. Cobb, the “father of US nematology,” pioneered agricultural nematology as a USDA scientist in the early 1900’s. The use of soil fumigation to reduce nematode populations and increase crop yields in the 1940’s (Carter) demonstrated that nematodes were significant crop pathogens and ushered in the “chemical era” for nematode management in production agriculture. For a review of the history of plant nematology see the book “General Nematology “by Armand Maggenti (1981); see also the nematode history web sites in Table 1. Today plant parasitic nematodes are recognized as major agricultural pathogens and are known to attack plants and cause crop losses throughout the world. Some estimates suggest they cause 77 billion dollars of damage worldwide each year (Sasser and Freckman 1987). As the full extent of damage caused by plant-parasitic nematodes is recognized by agricultural scientists, the study of the biology of plant-parasitic nematodes will become increasingly important.
Nematode Biology
The study of nematode biology has led to a dramatic increase in understanding of how all animals function. In fact, the bacterial-feeding nematode,
Caenorhabditis elegans, is one of the best-understood animals on earth. The fate of every cell in
C. elegans development has been carefully mapped. It was the first animal to have its DNA sequence completely deciphered, and it is amenable to detailed genetic analysis. The study of
C. elegans has led to new insights into the details of animal development, neurobiology, behavior, and has been of great value in biomedical research as well as in the understanding of nematode biology (Riddle et al. 1997). For more information on
C. elegans, also see the web sites listed in Table 1.
The reason nematodes are so useful for biological research is due to their simple anatomy and transparent bodies. Nematodes are simple animals, often only containing 1000 cells or less. Nematodes in all or part of their life cycle are worm-shaped (vermiform), although some species become swollen and rounded in later life stages (Figure 2). The basic body plan of a nematode is a tube within a tube (Figure 3). They have an outer skin or cuticle that is secreted from an inner hypodermis. The muscles are attached longitudinally to the nematode’s hypodermis, allowing them to move only in the dorsal ventral direction (snake-like movement). Inside the nematode there is an inner tube, the alimentary canal, which runs inside the nematode from head to tail. Between the alimentary canal and the body wall is fluid that provides pressure against the wall to maintain body shape and allow movement. At the head of a plant-parasitic nematode is a hollow mouth spear (like a hypodermic needle) called a stylet (Figure 3, 4). The nematode uses this stylet to puncture plant cells, to withdraw food and also to secrete protein and metabolites that aid the nematode in parasitizing the plant. The stylet is connected to the pharynx that, in turn, is connected to the intestine. The intestine ends at the rectum in the female nematode and the cloaca in the male. Attached to the pharynx are three - five salivary glands which produce secretions that may be emitted from the stylet and that assist the nematode in plant invasion and parasitism.
The nematode pharynx is muscular and specialized areas can contract and expand the esophageal lining. The expansion and contraction of the pharynx muscles allow the nematode to pump food into its intestine through its stylet or eject secretions from its salivary glands into and around plant cells. See nematode feeding film clips at the “nematodes feeding” web site in Table 1. In rhabditidan nematodes, the esophageal muscles are localized in a muscular organ called the metacorpus (Figure 3, 4). In enoplean nematodes, the esophageal muscles are more spread out and do not form a compact pumping organ.
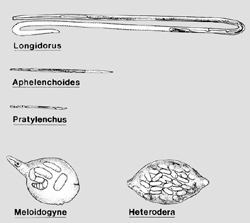 |
Figure 2. |
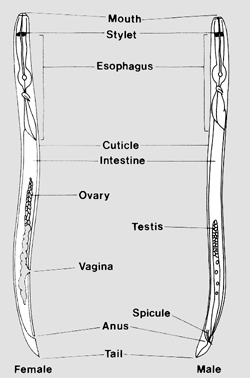 |
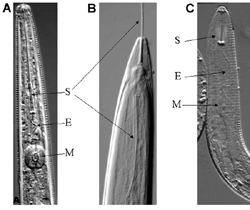 |
Figure 3. |
Figure 4. |
In the middle to posterior of the nematode are the reproductive organs. Nematode species often have both males and females, but it is not uncommon for plant nematodes to reproduce asexually by parthenogenesis. In females the reproductive organs are used as traits for identification because the number of ovaries and the position of the vulva in the female nematode's body are easily seen under the light microscope. Male nematodes have one or two testes and they are easily identified by the presence of spicules. Spicules are copulatory structures that are used during mating to guide the sperm into the vagina of the female nematode. See the film clip of soybean cyst nematodes mating at the “SCN mating” web site in Table 2. For a detailed review of nematode structure and function, see “The Structure of Nematodes” by Allan Bird (1991) or the nematode anatomy section of the USDA Nematology Lab web site in Table 1.
The common plant-parasitic nematode genera are fairly easy to identify to that level using a standard compound microscope. Identification of nematodes to the species level often requires detailed morphological analysis, growth of the nematode on different host plants, or DNA or isozyme analysis. Common morphological features used in nematode identification include the mouth cavity (presence or absence and shape of a stylet), the shape and overlap of the pharyngeal glands with the intestine, size and shape of the nematode body at the adult stage, size of the head, tail, and number and position of ovaries in the female. More subtle characters may include number of lines on the nematode’s cuticle or the presence or absence of pore-like sensory organs. An excellent guide to nematode identification is “Plant-Parasitic Nematodes: a Pictorial Key to Genera” by William F. Mai et al., 1996.
Plant-parasitic nematodes occur in all sizes and shapes. The typical nematode shape is a long and slender worm-like animal, but often the adult animals are swollen and no longer even resemble worms (Figure 2). Plant-parasitic nematodes range from 250 um to 12 mm in length, averaging 1 mm, to about 15-35 um in width. While nematodes may look dramatically different, they all share some common features. Nematodes often look segmented because of the numerous annulations (accordion-like transverse grooves) on the cuticle that allow the nematode to bend without kinking (Figure 4 A and C), but in fact nematodes are unsegmented and have no replication of body parts throughout the worm. Like most higher animals nematodes possess bilateral symmetry, but with a superimposed trilateral and hexalateral symmetry. Developmentally, nematodes are triploblastic, containing three body layers (ectoderm, mesoderm and endoderm) in the embryo. Most higher organisms are tripoblastic and have a coelom, a body cavity surrounded by mesoderm. Nematodes have a body cavity that is not totally surrounded by mesoderm, so they are pseudocoelomic.
Nematodes are evolutionarily related to insects, and one feature they have in common is the requirement to molt between juvenile stages. All nematodes undergo four molts from the juvenile to the adult phase of their life cycle (Figure 4B). They have four juvenile stages and an adult stage. In many nematodes the first molt usually occurs in the egg and it is the second-stage juvenile that hatches. While all nematodes undergo four molts, molting is not required for growth of the nematode as it is in insects because of the elasticity of the nematode cuticle. Nematodes do not have a skeleton, but they do have a hypodermis which functions as a flexible support for their muscles. Nematode muscles are arranged along the longitudinal axis of the worm, thus allowing the body of the nematode to move only in a sinusoidal (snake-like) motion. Nematodes have no defined respiratory or circulatory systems; they depend on diffusion of water, gasses and metabolites in and out of their semi-permeable body walls and internal transport by mixing of the pseudocoelomic fluid as the nematode moves. This lack of respiratory/ circulatory systems prevents nematodes from becoming larger in cross sectional area, but does not limit their length. The largest nematode found thus far was more than 7 meter long and 1 cm in diameter. Sorry, no giant nematodes; they are only in the movies. Nematodes have a sophisticated nervous system and sensory organs to help them find their host plant, to locate specific plant cell types, and to mate and reproduce.
Nematode Survival Strategies
One might think of soil as a safe environment, but to a microscopic nematode it is a hostile world filled with danger. A nematode must contend with voracious predators, changes in soil temperature and moisture, and the death of its host plant. For a nematode population to survive, it must be able to circumvent these obstacles. Nematodes evade these biotic and abiotic obstacles by employing a combination of behavioral and physiological survival strategies.
While all nematodes feed on other organisms, the soil is filled with bacteria, fungi and other nematodes that would gladly consume a nutrient-rich plant-parasitic nematode. In fact, the study of nematode predators and pathogens is an important area of study in nematology because nematode predators can be used (in theory) to control populations of plant-parasitic nematodes. While nematodes do possess a thick cuticle that may provide some protection from predation, this type of defense is easily breached by specialized nematode pathogens. The most common method plant nematodes use to evade predation is by living inside plant tissue or by limiting their mobility in the soil environment. By spending less time moving in the soil, a nematode can reduce its chance of "running into" a predator or pathogen. Some plant nematodes spend most of their time in the soil (ectoparasites) and others are mostly contained within the plant tissue (endoparasites). Nematodes that live inside plants have some degree of protection from predation, but they risk death if their host plant succumbs to disease. In contrast, nematodes that move from host to host reduce the risk of perishing with their host, but have a greater chance of encountering a predator or pathogen.
Nematode survival is not impacted only by biotic factors, but also by abiotic ones such as temperature and water availability. The onset of winter or the drying of the soil can be disastrous for a nematode. Interestingly, many nematodes are well adapted to abiotic stress and are capable of cryptobiosis (hidden life): the ability to enter a state of suspended metabolic activity during unfavorable environmental conditions (drying, heat, cold). While not all nematode are capable of cryptobiosis, the ones that are can often survive for years in a cryptobiotic state awaiting favorable conditions that will trigger their revival. The ability of nematodes to undergo cryptobiosis is one reason some nematode species are very difficult to eradicate from a field.
Although cryptobiosis is a useful survival strategy, not all nematodes can enter such a survival stage. Instead, some nematodes have adapted to feed upon many different plant species, thus avoiding the disaster of losing a host plant. Nematodes with a broad host range can feed upon weeds and wild plants making their eradication virtually impossible. By using a complex network of behavioral and biochemical protections nematodes are able to survive and prosper in what would seem to us to be hostile environments. For more information on nematode predators, see Poinar 1983 or the biocontrol web site listed in Table 1. For more information about cryptobiosis see Wharton, 1986.
Nematode Dissemination
While nematodes are motile animals, most are able to move no more than a meter through the soil within their lifetime. However, this lack of long distance crawling does not mean nematodes cannot rapidly spread from field to field. Farm equipment and even muddy shoes contaminated with nematode-infested soil can rapidly disperse nematodes. The movement of water during floods and irrigation can disperse nematodes over long distances. Likewise the movement of nematode infected plants, seeds, and bulbs can give nematodes international tickets to travel the world if plant quarantine officials are not careful. The ability of nematodes to form environmentally resistant stages makes their dissemination even easier, since dried nematodes can be blown with the wind or plant debris over large geographical regions. Even migrating birds are suspected to be able to carry nematodes along their flight paths, assisting the nematodes in their quest for new homes. Essentially any process that moves soil or plant tissue has the ability to disperse plant nematodes, making them difficult plant pathogens to quarantine. For a more detailed explanation of how the soybean cyst nematode has spread throughout the midwestern United States, see Noel, 1992.
Plant-Nematode Interactions
Nematodes feed on all parts of the plant, including roots, stems, leaves, flowers and seeds. Nematodes feed from plants in a variety of ways, but all use a specialized spear called a stylet. Note the differences in stylet length and shape (Figure 4). The size and shape of the stylet is used to classify nematodes and also can be used to infer their mode of feeding. All three nematodes in Figure 4 are ectoparasites, but
Belonolaimus and
Longidorus feed deep within the roots using their long stylets, while
Helicotylenchus feeds on the exterior of the root or partially burrows into the root to feed using its short stout stylet.
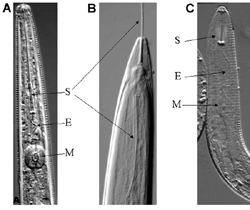 |
Figure 4. |
Often nematodes withdraw the contents of plant cells, killing them. When this type of feeding occurs, large lesions are formed in the plant tissue (Figure 13, 18). Some nematodes do not kill the plant cells they feed upon but “trick” the plant cells to enlarge and grow, thus producing one or more nutrient-rich feeding cells for the nematode. These feeding cells enable long term feeding associations, and form by repeated nuclear division in the absence of cell division (giant cells) or by the incorporation of adjacent cells into a syncytium formed by the breakdown of neighboring cell walls. See Figure 5 for a photomicrograph showing the feeding cells of
Meloidogyne (root-knot nematode). Collectively, nematodes can feed on almost any plant cell type, and form a variety of feeding cell types. The number of feeding cells can vary from one to a half dozen depending on the nematode species. For a view of how plant parasitic nematodes move and feed on plants see the film clips and the movies “Wormula” and “The Wormy” at the “nematode movies” web site in Table 1.
Many plant-parasitic nematodes feed on the roots of plants. The feeding process damages the plant's root system and reduces the plant's ability to absorb water and nutrients. Typical nematode damage symptoms are a reduction of root mass, a distortion of root structure and/or enlargement of the roots (Figure 6, 7, 8). Nematode damage of the plant's root system also provides an opportunity for other plant pathogens to invade the root and thus further weakens the plant. Direct damage to plant tissues by shoot-feeding nematodes includes reduced vigor, distortion of plant parts, and death of infected tissues depending upon the nematode species.
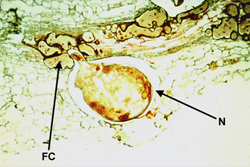 |
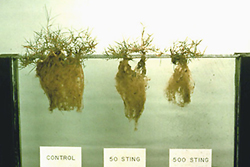 |
Figure 5. |
Figure 6. |
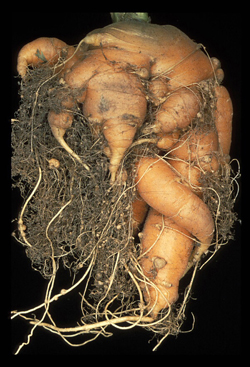 |
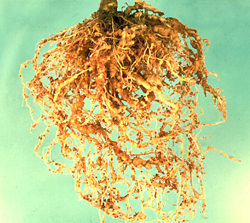 |
Figure 7. |
Figure 8. |
The aboveground symptoms of nematode damage to roots are relatively nondescript, including nutrient deficiency, incipient wilt, stunting, poor yield and sometimes plant death. Few diagnostic signs and symptoms of plant damage by nematodes exist except root galls, cysts, "nematode wool," and seed galls (see below). Thus, damage to crops by root-infesting nematodes often goes unnoticed by growers. Field patterns of nematode damage to roots begin in a small area and spreads radially from the initial infection site, often assisted by farm equipment. The only way to accurately diagnose nematode disease is to sample soil and plant material from suspected sites and extract nematodes for analysis. Nematodes are extracted from the soil by floating them in water to remove heavy soil particles and then catching the floating nematodes on sieves with fine pore sizes. Plant tissues infected with motile nematodes can be incubated in a
Baermann funnel or moist chambers to collect nematodes that will exit the tissues.
Most plant parasitic nematodes are soilborne root pathogens, but a few species feed primarily upon shoot tissues. The majority of plant parasitic nematode species are in the class Chromodorea, order Rhabditida (formerly placed in the order Tylenchida). There are seven major types of nematode feeding strategies used by plant parasitic nematodes (Table 2).
Ectoparasites: The first feeding type is the ectoparasitic mode, in which the nematode remains outside of the plant and uses its stylet to feed from the cells of the plant roots (Figure 9). Nematodes that use this strategy can graze on numerous plants, making it easier for them to switch hosts, but their added mobility makes them very susceptible to environmental fluctuations and predators. Ectoparasitic nematodes can have extremely long stylets, which assist them in feeding deep within the plant root on nutrient rich plant cells (Figure 4, 10). Some of these nematodes induce the plant to form an enlarged cell(s) that the nematode feeds from for an extended period of time. Note, in all life cycle diagrams in this article the abbreviation J=juvenile and the number refers to the stage of the nematode and M=molt and refers to how many molts the nematode has completed.
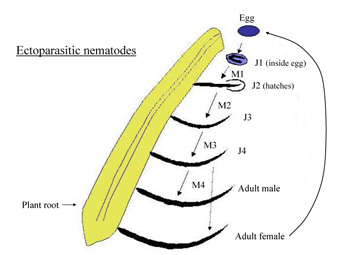 |
Figure 9. |
The above life cycle is typical for a nematode in the class Enoplea (Figure 9), but most nematodes in the class Chromadorea undergo their first molt in the egg and hatch as J2. All motile nematode stages are capable of feeding from the plant. The nematodes feed, undergo four molts into adults, mate and lay eggs. Some nematodes that use this feeding strategy can form terminal galls in the roots and cause severe stunting of the root system. An example of an ectoparasite is
Xiphinema (dagger nematode), shown below in Figure 10, feeding from a fig root. This enoplean nematode is particularly problematic because it can harbor and transmit plant viruses. Only ectoparasitic nematodes in the class Enoplea transmit viruses, but because of the virus transmission, this type of nematode in low numbers can still be very damaging to plants (Fisher and Raski, 1967; Hewitt et al. 1958).
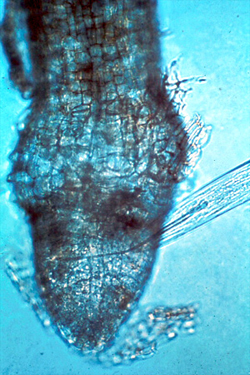 |
Figure 10. |
Semi-endoparasites: Nematodes that feed as semi-endoparasites are able to partially penetrate the plant and feed at some point in their life cycle (Figure 11). Usually the head of the nematode penetrates into the root and allows the nematode to form a permanent feeding cell. These nematodes swell and do not move once they have entered into the endoparasitic phase of their life cycle. By giving up their mobility, the nematodes risk death if their host plant dies, but they also benefit from forming a permanent feed site, which increases their nutrient uptake and reproductive potential. A typical nematode with this life cycle is
Rotylenchulus reniformis, the reniform (kidney-shaped) nematode. This nematode hatches from the egg as a J2, then quickly molts in the soil to the adult stage without feeding. The anterior end of an adult female enters the plant root and forms a feeding cell. After mating, the female lays its eggs outside of the root in a gelatinous egg mass Maggenti (1981). Another nematode with a similar feeding strategy is
Tylenchulus semipenetrans, the citrus nematode, although the juvenile stages of this nematode do feed as ectoparasites. As is common in biological systems, it is often difficult to precisely classify animals due to variation in their behavior. True to this rule, several species of ectoparasitic nematodes (e.g.
Helicotylenchus) are also capable of partially penetrating the root and feeding. However, we do not classify these nematodes as semi-endoparasites because they do not exhibit a consistent endoparasitic feeding behavior.
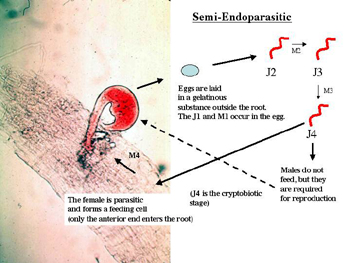 |
Figure 11. |
Migratory endoparasites: Migratory endoparasitic nematodes spend much of their time migrating through root tissues destructively feeding on plant cells (Figure 12, 13). These nematodes cause massive plant tissue necrosis because of their migration and feeding. When they feed from the plant, they simply suck out the plant cell cytoplasm using their stylet, killing the plant cell and moving ahead of the lesion. They make no permanent feeding cells. In a typical life cycle, the nematode hatches from the egg as a second-stage juvenile and starts feeding on the plant. The nematodes feed, molt and reproduce primarily within the plant tissue. All motile stages are capable of feeding from the plant and they are able to move into the soil in search of new roots to invade. Because these nematodes create extensive wounds in the plant root, secondary infection by bacteria and fungi can often occur, further damaging the root system (Zunke 1991). Below is a typical life cycle for a migratory endoparasite. Examples of migratory endoparasitic nematodes are
Pratylenchus (lesion nematode),
Radopholus (burrowing nematodes) and
Hirschmanniella (rice root nematode).
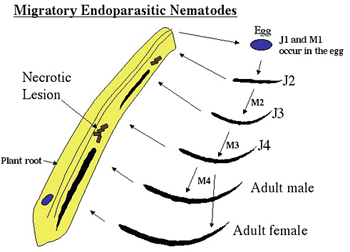 |
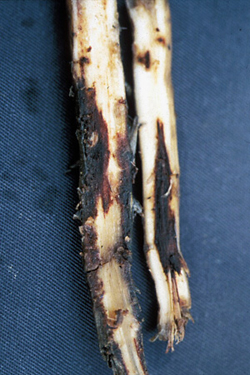 |
Figure 12. |
Figure 13. |
Sedentary endoparasites: The most damaging nematodes in the world have a sedentary endoparasitic life style. The two main nematodes in this group are the cyst nematodes (Heterodera and
Globodera) and the root-knot nematodes (Meloidogyne). In these nematodes, the J2 invades the plant near the tip of a root and migrates through the tissue to the developing vascular cells. These nematodes are completely embedded in the root during their initial stages of development, but later the cyst nematodes protrude from the root. The J2 nematodes inject secretions into and around the plant cells to stimulate the formation of large feeder cell(s), which they non-destructively feed on throughout their life cycle. Feeding cells of root-knot nematodes (giant cells) form by repeated nuclear division in the absence of cell division. Feeding cells of cyst nematodes form by the incorporation of neighboring cells into a syncytium formed by the breakdown of neighboring cell walls. Once the feeding cells are formed, the juvenile nematode rapidly becomes sedentary because their somatic muscles atrophy. The juveniles feed, enlarge and molt three times to the adult stage. The large feeding cells formed by these nematodes plug the vascular tissue of the plant making it susceptible to water stress. Female sedentary endoparasites enlarge considerably into a saclike shape and are capable of laying large numbers of eggs. Eggs are typically laid outside the nematode in a gelatinous egg mass, but in cyst nematodes most eggs are retained inside the female body. Both types of nematode have the same basic feeding strategy, but many cyst nematodes have an obligate sexual cycle, whereas the most common species of root-knot nematodes reproduce largely by parthenogenesis.
Below, the life cycle of the soybean cyst nematode (Heterodera glycines) is described (>Figure 14, 15, 16). The cyst nematodes are extremely problematic because they have the ability to persist for a long period of time in a field. Their remarkable persistence is due to their ability to produce a cyst, which is the hardened dead body of the female nematode that surrounds the eggs. The eggs in the cyst are in a dormant state and they hatch slowly over years in the field, usually in response to signals from host roots. Having a resistant or dormant nematode stage enables nematodes to survive non-optimal conditions. Resistant stages also aid the nematode in dispersal. While the root-knot nematodes do not have an environmentally resistant stage, they do possess a broad host range that enables them to persist on an alternate host. The use of environmentally resistant nematode stages and broad host ranges are two key strategies plant nematodes use to persist in the environment. For further information about the soybean cyst nematode see the "Biology and management of the soybean cyst nematode" edited by R.D. Riggs and J.A. Wrather. For further information about the root-knot nematode see "An advanced Treatise on
Meloidogyne" edited by J.N. Sasser and C.C. Carter.
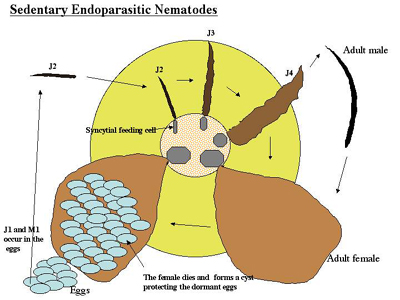 |
Figure 14. |
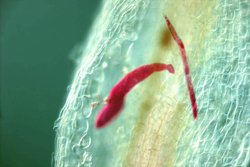 |
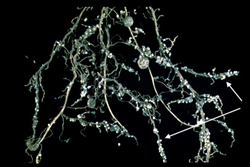 |
Figure 15. |
Figure 16. |
Stem and bulb nematodes: Stem and bulb nematodes (Ditylenchus spp.) are, as their name suggests, nematodes that attack the upper and lower parts of plants (Figure 17). They use water films to migrate up the stem of the plant and therefore are more damaging under wet conditions. The infectious stage of the stem and bulb nematodes is the fourth stage juvenile. This stage often enters emerging plant tissues below ground, but can crawl up stems in a film of water and enter shoots via buds, petioles, or stomata. Once in the host plant, they destructively feed as migratory endoparasites, molt into adults and reproduce. The nematodes hatch from the egg as J2 and continue to feed, molt and reproduce, extensively macerating and distorting the plant tissue (Figure 18). Once the plant is destroyed or winter arrives, the stem and bulb nematode juveniles arrest their development at the environmentally resistant J4 stage and overwinter. Fluffy masses of dried (cryptobiotic)
Ditylenchus can be seen on the surface of bulbs and are known as "nematode wool." Once environmental conditions are favorable, the cryptobiotic J4 become active and their life cycle resumes (Hooper 1972).
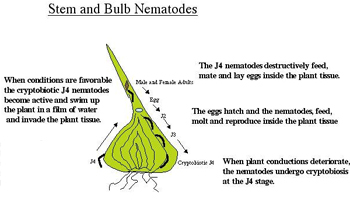 |
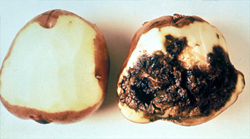 |
Figure 17. |
Figure 18. |
Bursaphelenchus xylophilus (pine wood nematode), a nematode that infects pine trees, has a different and fascinating life cycle (Figure 19). Resistant stages of these nematodes are carried into pine tree in the tracheae of bark beetles. Once in the tree the nematodes are released from the beetle, and they migrate through the resin canals of the tree. The pine wood nematode destructively feeds from cells in the resin canals, clogging them and the tree's vascular tissue causing pine wilt disease. The nematodes feed, molt and sexually reproduce at a highly accelerated rate. The population of pine wood nematodes builds up rapidly and quickly kills the host tree. The nematodes are able to feed on fungi in the tree after the plant cells are dead. The nematodes survive the winter as J3s and are attracted to beetle larvae. In the spring the nematodes molt to the resistant J4 stage and invade the beetle to be dispersed to neighboring trees. The ability of the nematode to be dispersed by an insect allows them to spread rapidly from tree to tree, a process which can quickly destroy a forest (Mamiya 1983). This nematode is thought to be indigenous to the Unitied States where all of the native trees are resistant to the damaging effects; however in Asia and Europe, the pine tree species are very susceptible, which has resulted in the death of millions of trees each year.
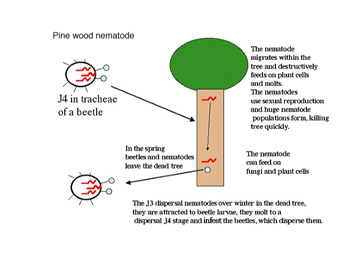 |
Figure 19. |
Seed gall nematodes: Seed gall nematodes (Anguina spp.) were the first plant-parasitic nematodes to be described in the scientific literature in 1743 (Figure 20). These nematodes migrate as J2s in water films to the leaves of plants where they feed as ectoparasites at the tips, causing distortion of the leaves. Once the plant starts to flower the J2 penetrates the floral primordia and starts to feed on the developing seed. Once in the seed, the nematode undergoes its molts, continues to feed, and eventually kills the seed to form a blackened "cockle" (seed gall). The adults sexually reproduce, the eggs hatch as J1 and then quickly molt into a J2 survival stage. The environmentally resistant J2 desiccates with the seed gall and overwinters. The nematodes in the seed gall can survive for 30 years if kept in a dry location. When proper moisture and temperature conditions arise, the cryptobiotic J2 becomes active and starts the life cycle over again (Southey 1972).
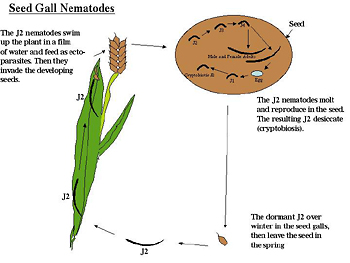 |
Figure 20. |
Foliar nematodes: Foliar nematodes are in the genus
Aphelenchoides. The adult nematodes migrate in water films on the stems to the leaves of their host plant and penetrate the leaves through natural openings (stomata) (Figure 21). Once in the leaves the nematodes migrate, destructively feed, molt and lay eggs. The feeding activity of the nematodes causes characteristic interveinal chlorosis and necrosis of the leaf, ultimately killing it. The nematodes are able to move from leaf to leaf if the proper (moist) environmental conditions exist and can severely damage a plant. In the winter the adult nematodes persist in the dead leaves until favorable conditions arise in the spring. If the dead, nematode-infested leaves are moved or blown around this will help disperse the nematode near new host plants (Hesling and Wallace 1961).
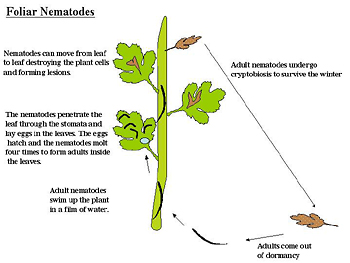 |
Figure 21. |
Plant Nematode Control
There are several methods commonly used to control plant-parasitic nematodes. These methods can be divided in to three main types: biological control, cultural control and chemical control. The most practical form of biological control is the use of nematode-resistant plants. In this control method, plant breeders cross natural nematode resistance genes into cultivated plant species to improve their resistance to nematodes. The benefit of this method is that it is a very inexpensive way for growers to control their nematode problems. The main disadvantage is that it takes years to screen for resistant plant varieties and more time to breed resistance traits into commercial varieties. Further complications are that natural sources of nematode resistance do not exist for all cultivated species and some species of nematodes are able to grow on resistant plants. However, when "good" resistant plants are available, they are an effective method of nematode control. To find a list of plants resistant to nematodes see the plant host database web site in the Department of Nematology at the University of California at Davis (Table 2). Biotechnology has the potential to produce nematode resistance genes that would be effective against many types of nematodes and would function in many different plant species. However, at the present time there are no plants with bioengineered nematode resistance available for commercial use.
Other biological control methods use natural predators or pathogens of nematodes. While biological control agents kill nematodes in controlled laboratory settings, implementation of biological control methods in the field is not done due to the expense and difficulty of growing large amounts of nematode pathogens. However, if some of the difficulties associated with growing nematode pathogens can be overcome, this may be a promising method of nematode control in the future.
Crop rotation with a non-host plant is a very effective method to limit nematode growth. Typically, a cropping system is devised that selects plants that nematodes can and cannot grow on. These plants are grown in alternate years and the problematic nematode population decreases dramatically, below damage threshold levels, in the years that the non-host is grown. This can be an effective method if a producer has the choice of several different crops that can be grown and if the problematic nematode does not have a broad host range or survive in the soil in a cryptobiotic state for long periods of time. See the UC Davis nematode databases in Table 2 to ascertain the host range of various plant parasitic nematodes.
For the past 50 years nematodes have been effectively controlled using chemical nematicides. These are inexpensive chemicals that effectively kill nematodes in soil. There are two types of nematicides, soil fumigants (gas) and non-fumigants (liquid or solid). Soil fumigants became popular because they did not rely on alternative host crops for rotation; they drastically reduced nematode populations in the soil, and were cost effective for most crops. Most fumigant nematicides have been banned by the EPA as environmental toxins with the exception of 1,3 dichloropropene (Telone II), chloropicrin (tear gas), and dazomet (Basamid). The multipurpose soil fumigant methyl bromide also provides excellent reduction of soil nematode populations, but methyl bromide was largely discontinued in 2005. Non-fumigant nematicides such as fenamiphos (Nemacur) and aldicarb (Temik) are based upon the same kinds of active ingredients as many insecticides (i.e. nerve poisons) and can be applied in liquid or granular formulations. While non-fumigant nematicides reduce nematode populations, their effectiveness is not as consistent as that of fumigant nematicides. The EPA is also restricting the use of non-fumigant nematicides. Since nematicides are expensive to develop, new ones are rarely released on the market today. While nematicides are effective in controlling nematodes, they are only practical for use on high-value crops.
Plant nematodes are not typically controlled using just one method mentioned above, but instead they are managed using a combination of methods in an integrated pest management system. For more detailed information about nematode control see "Plant Nematode Control" by A.G. Whitehead 1998 or the web site for chemical methods of nematode control in Table 1.
Table 2. Summary of Plant Parasitic Nematode Feeding Strategies |
Feeding Strategy |
Example Genera |
Order |
Infective Stage |
Resistant Stage |
Notes |
Ectoparasite | Belonolaimus Xiphenema Trichodorus | Rhabditida Dorylaimida Triplonchida | J2-adult J2-adult J2-adult | |
Vector viruses Vector viruses |
Semi-Endoparasites | Rotylenchulus Tylenchulus | Rhabditida Rhabditida | J4 J2 | J4 J2 | |
Migratory Endoparasites | Pratylenchus Radopholus | Rhabditida Rhabditida | J2-adult | * | |
Sedentary Endoparasites | Meloidogyne Heterodera Naccobus | Rhabditida Rhabditida Rhabditida | J2 J2 J2 | Egg/cyst | |
Stem and Bulb Nematodes | Bursaphelenchus Ditylenchus | Rhabditida Rhabditida | J4 J4 | J3 J4 | J4 vectored by insects |
Seed Gall Nematodes | Anguina | Rhabditida | J2 | J2 | |
Foliar Nematodes | Aphelenchoides | Rhabditida | J2-adult | Adult | |
* eggs, all juvenile stages and adults can survive the winter, but not egg producing females.
Other APS Education Center nematode links
K-12 Exercise Tylka, G.L. and C.A. Jasalavich. 2001. Nematodes: Plant-Parasitic Worms.
References
Barker, K.R., G.A. Pederson, and G.L. Windham. 1998. Plant and Nematode Interactions. ASA, CSSA, and SSSA, Madison, WI.
Bird, A.F. and J. Bird. 1991. The Structure of Nematodes, Second edition.Academic Press Inc. London.
Blaxter, M.L., P. De Ley, J.R. Garey, L.X. Liu, P. Scheldeman, A. Vierstraete, J.R. Vanfleteren, L.Y. Mackey, M. Dorris, L.M. Frisse, J.T. Vida, K.W. Thomas, K.W. 1998. A molecular evolutionary framework for the phylum nematoda. Nature 392:71-75.
Campbell, J.F. and H.K. Kaya. 1999. How and why a parasitic nematode jumps. Nature 397: 485-486.
Chen, Z.X., and D.W. Dickson. 2004. Nematology: Advances and Perspectives Vol. 1: Nematode Morphology, Physiology and Ecology. CABI:Walllingford.
Chen, Z.X., S.Y. Chen, and D.W. Dickson. 2004. Nematology: Advances and Perspectives Vol. II: Nematode Management and Utilization. CABI:Wallingford.
Fisher, J.M. and D.J. Raski. 1967. Feeding of
Xiphinema index and
X. diversicaudatum. Proceeding of the Helminthological Society of Washington 34:68-72.
Hewitt, W.B., D.J. Raski, and A.C. Goheen. 1958. Nematode vector of soil-borne fanleaf virus of grape vine. Phytopathology 48:586-595.
Hesling, J.J. and H.R. Wallace, H.R. 1961. Observations on the biology of chrysanthemum eelworm Aphelenchoides ritzemabosi (Schwartz) Steiner in florists chrysanthemum. I. Spread of eelworm infestation. Annals of Applied Biology 49:195-209.
Hooper, D.J. 1972.
Ditylenchus dipssaci. Commonwealth Institute of Helminthology Descriptions of Plant Parasitic Nematodes, Set 1, No. 14, St. Albans, England.
Lee, D.L. 2002. The Biology of Nematodes. Tayor & Francis; New York
Maggenti, A. 1981.General Nematology. Springer-Verlag, New York, NY.
Mai, W.F., P.G. Mullin, H.H. Lyon, K. Loeffler. 1996. Plant-parasitic nematodes: a pictorial key to genera. Cornell University Press, Ithaca, NY.
Mamiya, Y. 1983. Pathology of the pine wilt disease caused by
Bursaphelenchus xylophilus. Annual Review of Phytopathology 21: 201-220.
Manum, S.B., M.N. Bose, R.T. Sayer, R.T., S. Bostrom. 1994. A nematode (Captivonema-Cretacea Gen ET SP-N) preserved in a clitellate cocoon wall from the Early Cretaceous. Zoologica Scripta. 23: 27-31.
Perry, R.N. and M. Moens. 2006. Plant Nematology. CABI: Wallingford.
Noel, G.R. 1992. History, Distribution, and Economics. Pages 1-13 in: Biology and Management of the Soybean Cyst Nematode. R.D. Riggs and J.A. Wrather, eds. American Phytopathological Society, St. Paul, MN.
Poinar, G.O., A. Acra, and F. Acra. 1994. Earliest fossil nematode (Mermithidae) in Cretaceous Lebanese amber. Fundamental and Applied Nematology 17:475-477.
Poinar, G.O. 1983. The Natural History of Nematodes. Prentice Hall, Englewood Cliffs, NJ.
Powers, T.O., T.S. Harris, and B.C. Hyman, B.C. 1993. Mitochondrial DNA sequence divergence among
Meliodogyne incognita,
Romanomermis culicivorax,
Ascaris suum, and
Caenorhabditis elegans. Journal of Nematology 25:564-572.
Riddle, D.L., T. Blumenthal, B.J. Meyer, J.R. Priess (eds). 1997.
C. elegans II. Cold Spring Harbor Monograph Series 33. Cold Spring Harbor Laboratory Press. Plainview, NY.
Riggs, R.D., and J.A. Wrather. 1992. Biology and Management of the Soybean Cyst Nematode. American Phytopathological Society, St. Paul, MN.
Sasser, J.N. and C.C. Carter. 1985. An advanced Treatise on
Meloidogyne. North Carolina State University Graphics, Raleigh, NC.
Sasser, J.N. and D.W. Freckman. 1987. A world perspective on nematology: the role of the society. Pages 7-14 in: Vistas on Nematology, J.A. Veech and D.W. Dickson, eds. Society of Nematologists, Inc. Hyattsville, MD.
Siddiqi, M.R., 2000. Tylenchida. CABI: Wallingford.
Shurtleff, M.C., and C.W. Averre. 2000. Diagnosing Plant Diseases Caused by Nematodes. APS Press: Minneapolis
Southey, J.F. 1972.
Anguina tritici. Commonwealth Institute of Helminthology Descriptions of Plant Parasitic Nematodes, Set 1, No. 13, pp.1-4. St. Albans, England.
Starr, J.L., J. Bridge, and R. Cook. 2002. Plant resistance to parasitic nematodes. Oxford University Press; Oxford
Thomas, K.W., J.T. Vida, L.M. Frisse, M. Mundo, J.G. Baldwin. 1997. DNA sequence from formalin-fixed nematodes: integrating molecular and morphological approaches to taxonomy. Journal of Nematology 29:250-254.
Viglierchio, D.R. 1991. The World of Nematodes. AgAccess, Davis, CA.
Wang, D.Y.-C., S. Kumar, and B.S. Hedges. 1999. Divergence time estimates for the early history of animal phyla and the origin of plants, animals and fungi. Proceedings of the Royal Society of London B 266: 163-171..
Wharton, D.A. 1986. A Functional Biology of Nematodes. The John Hopkins University Press, Baltimore, MD.
Whitehead, A.G. 1998. Plant Nematode Control. CAB International, New York. NY.
Zunke, U. 1991. Observations on the invasion and endoparasitic behavior of the root lesion nematode
Pratylenchus penetrans. Journal of Nematology 22:309-320.