Themis J. Michailides
Yong Luo
Zhonghua Ma
David P. Morgan
Department of Plant Pathology, University of California Davis,
Kearney Agricultural Center, Parlier, CA 93648
Corresponding authors: T. J. Michailides and Y. Luo
Michailides, T., Luo, Y., Ma, Z., and Morgan, D.P. 2007. Brown Rot of Dried Plum in California: New Insights on an Old Disease. Online. APSnet Features. doi: 10.1094/APSnetFeature-2007-0307
Introduction
Production of dried plum (prune, Prunus domestica L.) in California is a 100-million-dollar industry. Of more than 300,000 acres of stone fruits grown in California, 75,000 acres include dried plums grown from the southern San Joaquin Valley to the northern Sacramento Valley. Counties with significant dried plum acreage include Tulare, Fresno, Madera, Merced, Yolo, Yuba, Sutter, Butte, Colusa, Glenn, and Tehama. California produces approximately 99% of the United States dried plum supply and 60% of the world supply. California dried plums are exported to more than 50 countries including Japan, Germany, United Kingdom, Italy, China, Belgium, Mexico, Australia, and to some other smaller international markets. ‘French’ is the major cultivar of dried plum commercially used in California. The bloom season of this cultivar usually begins around March 15 (green tip stage) to April 15 (fruit set stage) (Fig. 1) (45). To achieve a desirable increased fruit size and drying ratios, California growers usually thin the fruit by shaking the trees so that the fruit remaining on the tree become large. Other reasons for thinning dried plums are to avoid all the problems associated with overcropping, such as limb breakage, excessive dry-away, and severe potassium deficiency (tree dieback). The harvest season usually begins in late August to early September depending on weather and orchard conditions. The majority of the dried plum crop produced is dehydrated immediately after harvest (preferably within 24 hours) in dehydrators (Fig. 2) while a small portion of the crop is now sold as fresh. Dehydrated fruit are kept in storage and upon market orders, they are rehydrated, pitted, and sent to domestic or international markets.
 A |
|
 B |
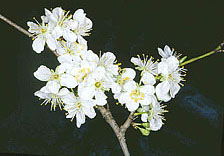 C |
 D |
 E |
Fig. 1. Phenological stages of bloom development of dried plum (Prunus domestica): (A) green tip; (B) popcorn stage; (C) full bloom; (D) petal fall; and (E) chuck split stage.
|
 A |
|
 B |
|
|
Fig. 2. Prunes after harvest are placed (A) in wooden trays; (B) trays with prunes after dehydration. |
|
Causal Pathogens
Brown rot is an economically and ecologically important disease of dried plum caused by two ascomycete fungi, Monilinia fructicola (G. Wint.) Honey and M. laxa Aderhold & Ruhland (8). These pathogens can cause blossom blight in spring, rot of green fruit in mid season, and decay of mature fruit in late season (3,8,22,46). M. laxa usually causes more severe blossom and twig blights, while M. fructicola causes more severe fruit decay. In addition, M. laxa is common on almonds and apricots, while M. fructicola is currently the dominant pathogen causing brown rot of peaches, nectarines, and plums. Major changes in the populations of Monilinia spp. in dried plum orchards have occurred over the years. In the past, M. laxa was the most common brown rot fungus found in dried plum orchards in California. Now the pathogen population has shifted and M. fructicola is the more prevalent species (39). A partial explanation of the shift of the two species in dried plum orchards is the fact that M. laxa isolates have been sensitive to benzimidazoles fungicides while those of M. fructicola developed resistance to these fungicides and predominated in orchards.
Distinguishing Monilinia fructicola from M. laxa based on morphological characteristics of their spores is not easy. The colonies of M. fructicola on potato dextrose agar acidified with lactic acid (at 2.6 ml of 25% lactic acid per liter) may be fluffy, while colonies of M. laxa are relatively clear with less mycelium density and wedged (loboid) margins (Fig. 3). The development of M. fructicola-specific primers have made identification and diagnosis of the brown rot fungi easier and more efficient than it used to be. A primer developed based on the small-subunit rDNA gene was used to distinguish M. fructicola from M. laxa and M. fructigena (13). A sequence of the rDNA internal transcribed spacer (ITS) has also been used in PCR to distinguish the above three species (19). The sequence of a random amplified polymorphic DNA (RAPD) region (12) and the species-specific repetitive sequences (6) were used to develop the M. fructicola-specific primers. These primers provide the potential to detect M. fructicola in plant material with visible symptoms and/or latent infections.
|
Fig. 3. Cultures of Monilinia fructicola (right plate) contrasted with M. laxa (left plate) on acidified potato dextrose agar after 10 days at 25°C. |
|
Although widespread outbreaks of brown rot of dried plum over the entire state rarely occur, disease development on an orchard scale frequently depends on the pathogen’s inoculum potential, microclimatic conditions, and cultural practices. For instance, in 1993 and 1998, blossom blight and fruit rot of dried plums were severe in California, and the estimated losses reached up to 25% of the crop. In normal years, however, the incidence of fruit rot can vary from orchard to orchard and range from 0 to 10%, depending on the specific orchard conditions. Based on recent surveys of dried plum and other stone fruit growers, brown rot is still the number one disease of concern.
Symptoms
The symptoms caused by the two fungi generally are similar. In spring, infected flowers wither, spurs and leaves collapse, and a small canker may form in the subtending shoot (Fig. 4). If girdled, the shoot dies. Dead flowers, and sometimes leaves or dead shoots, develop shortly after bloom. In years with continuous rains in the spring, even leaves of dried plum can also be infected by M. fructicola (Fig. 5). Leaf lesions are brown, water soaked, and under favorable environmental conditions, sporulation of the pathogen can develop on the surface. Most blossom parts of dried plum, including sepals, petals, anthers, and stigmas, are susceptible to the pathogen. Infected blossoms collapse or "blight" within one week, followed by wilting of terminal leaves, and blighting of the whole twig. Gum may exude from the infected shoots and gray to brown tufts of spores produced in sporodochia often are found on infected tissues. Gray- to tan-colored tufts of spores emerge on infected blossoms and blighted twigs. Infected blossom parts (such as shucks) may cling to enlarging fruit, and with moisture, they tend to produce spores of the pathogen, thus serving as sources of inoculum for infection of the developing green fruit. Infections of green fruit begin as soft water-soaked, dark areas. Rot first develops in clustered fruit (Fig. 6), in fruit contact spots, and insect- or wind-damaged fruit. Clustered fruit have characteristics that are more favorable for disease development. For instance, fruit-to-fruit contact surfaces have microcracks, thinner cuticles, and favorable micro-environmental conditions, all predisposing fruit to infection (35).
 A |
|
 B |
|
 F |
|
 G |
|
Fig. 4. Symptoms of (A and B) blossom blight; (C and D) blossom and shoot blights resulting in canker; (E) decay of immature fruit; (F) rot of mature dried plum fruit caused by M. fructicola; and (G) infected fruit drying on the tree become shriveled.
|
 A |
|
 B |
|
 C |
Fig. 5. (A) Visible latent (quiescent) infections on a leaf; (B) water soaked lesions caused by M. fructicola on leaves of dried plum; and (C) one leaf with sporulation on the lesion.
|
Fig. 6. Decay of mature dried plum fruit in a cluster caused by M. fructicola. |
|
Latency is expressed in two forms in dried plum. Quiescent infections are seen as small black specks on the fruit surface (visible latent infections) while "true" latent infections are invisible (invisible latent infections) (47) (Fig. 7). Depending on weather conditions, some of these infections resume growth in summer and decay either green or mature fruit. Brown rot infections of mature fruit begin as soft water-soaked areas that turn dark brown whose surface eventually will be covered with sporodochia of Monilinia spp., usually emerging through lenticels or cracks on the fruit skin. Clustering of fruit, hail, insect, and bird wounds predispose dried plum fruits to brown rot (35). Russet scab and wind scab can also predispose fruit to infection by the pathogen (33,34). Infected fruit shrivel and dry on the trees and become what growers call "mummies". Often, decay moves from a fruit to the surrounding fruit in clusters and several brown-rotted fruit stick together in clumps ["mummy clusters" (Fig. 8)]. Other times, individually decayed fruit hang singly on the tree and referred as "single mummies". During fall and winter when the rains begin in California, some of these "mummies" will drop on to the ground while a number of them will hang on to the trees until next spring.
 A |
|
 B |
|
 C |
|
Fig. 7. Visible latent (quiescent) infections of (A and B) green dried plum fruit in an early developmental stage as contrasted to (C) those developed to decay caused by M. fructicola.
|
Fig. 8. A cluster of 21 mummified fruit ("cluster mummy") resulted from dried plum fruit infected by M. fructicola. |
|
Inoculum
The major source of inoculum in dried plum orchards for blossom and young fruit infection are mummies that produce conidia and ascospores in apothecia. The major source of inoculum that results in fruit infection in mid and late season is the mummified fruit on trees or thinned fruit on the ground. Ascospores of M. fructicola, produced from apothecia that develop from mummies on the orchard floor (Fig. 9), are one of the spore sources that can infect blossoms and cause blight in the spring. However, ascospores and apothecia of M. laxa have not been observed in California orchards. Mummified fruit are pseudosclerotia (part fungus and part fruit tissues) and form from shriveled infected ripe fruit, although occasionally infected green fruit can also dry on the tree and produce mummies. Mummified fruit that fall to the ground can produce apothecia in spring when conditions in the proximity of the mummies are favorable. For instance, areas where water accumulates and soil remains wet longer (indicated by the development of green algae) can provide such types of favorable conditions (Fig. 9c). Small changes in humidity in the proximity of mature apothecia cause an automatic discharge of ascospores. Even movements of small animals can trigger ascospore discharges. A mummified fruit can produce from one up to 35 apothecia within one to two weeks. Each apothecium can produce from 2 to more than 5 million ascospores (17). Subsequent apothecia produced by a mummified fruit are smaller in size (15). Discharge of ascospores is temperature dependent and can continue for an average of 4 days. The optimum temperature for ascospore discharge and ascospore germination is 15°C (59°F). Discharge of ascospores will be extended when temperatures are below 10°C (50°F) and be shorter when temperatures are above 15°C (59°F) (17). Interestingly, conditions that are required for breaking dormancy of flower buds and those favoring maturation of apothecia coincide with bloom of dried plum trees, thus invisible clouds of ascospores are carried on to susceptible tissues and cause brown rot disease. This is considered a primary infection and an important part of the initiation of the disease cycle in spring for M. fructicola under California conditions.
 A |
|
 B |
 C |
|
 D |
|
 E |
|
Fig. 9. Various apothecia of M. fructicola from mummies of dried plums: (A) apothecia developed from mummies buried under the soil surface (long stipes); (B) apothecia from a mummy found on the surface of the soil (short stipes); (C) multiple apothecia from a mummy partially buried (dark color apothecia are a few days old; light color apothecia are young — notice algae on the surface of soil, suggesting locations in orchards where water stays long on the surface after a rain); (D) apothecia from mummies under dense cover crop/weeds (long stipes); and (E) apothecia triggered to release ascospores using a cotton swab soaked in 85% acetic acid.
Conidia are another source for blossom infection in spring. Most conidia on mummies left over from sporulation of the pathogen in the previous season are not viable due to dryness of mummified fruit, contamination by or competition for available tissues with other fungal species, as well as extreme orchard microclimatic environments. Frequently, some mummified fruit, when wetted and temperatures are favorable, produce a new crop of viable conidia that can serve as inoculum in the spring (Fig. 10). Although the incidence of mummified fruit on trees with viable M. fructicola producing conidia in the spring can reach up to 30% in plum orchards, this incidence is much lower in dried plum orchards (T. Michailides and Y. Luo, unpublished data). Moreover, some mummified fruit on the orchard floor can produce conidia in the spring in some orchards under very favorable conditions.
|
Fig. 10. New conidia produced by M. fructicola on dried plum mummies. |
|
Which kind of inoculum, ascospores or conidia, is the major source of blossom infections is dependent upon the conditions specific to an orchard. Because conventional methods cannot answer the question about the reproductive modes of M. fructicola in orchards in the spring, a molecular approach was developed in a recent study (Y. Luo et al., unpublished data). Two populations of M. fructicola isolates collected from blossoms in a plum and a dried plum orchard, respectively, were analyzed by using the microsatellite primed- (MP-) PCR for all DNA phenotypes showing unique band patterns. The length of the most parsimonious tree for these isolates was obtained using the heuristic search option with 1000 random addition replicates with tree bisection reconnection to produce a distribution of tree length. The null hypothesis is that the population sampled undergoes recombination. If the length of the observed tree is significantly shorter than the average length of the trees from the recombination data, the hypothesis can be rejected to conclude that the isolates sampled came from a population with clonal reproduction mode. Otherwise, the sampled isolates should likely have come from a population with recombining reproduction (7). The analysis indicated that the isolates collected from the plum orchard derived from a population with recombining reproduction (ascospores), while the isolates collected from the dried plum orchard derived from a population with major clonal reproduction (conidia).
Using microsatellite primed- (MP-) PCR and molecular analysis, the genetic structure within a M. fructicola population was found to be stable during a single growing season (Luo et al., unpublished data). The genetic diversity values (Hs) (41) were less than 0.3 for sampled populations from a plum orchard and a dried plum orchard. The very high levels of genetic identity (Gi) among isolates collected at different times of a season in the same orchard indicates that, once established, over the course of a single growing season, little genetic changes occur within the populations.
When spore inoculum was quantified by using Burkard spore traps placed in dried plum orchards, very high daily spore densities of Monilinia in the air were found during bloom. But the spore density decreased after May and remained at very low levels during the rest of the season (30, Y. Luo et al., unpublished data). However, when thinned fruit were present on the floor of the orchards daily, density of spore inoculum increased (Figs. 11 and 12). A similar situation was also recorded in nectarine orchards in California (16). The amount of conidia produced from thinned fruit increased exponentially with increasing water content of thinned fruit (or fruit dryness) and ranged from 2 to 8 × 107 spores per fruit per day (25). The duration of sporulation in days increased linearly with increasing water content of thinned fruit with an average of less than 10 days. The proportion of thinned fruit with sporulation decreased linearly with time after fruit thinning; it appears that the speed by which thinned fruit are drying on the orchard floor is critical in inoculum production dynamics during the season. Since the incidence of fruit with latent infection increases linearly with increasing inoculum concentration (24), spore density in the orchard air could also relate quantitatively to the incidence of fruit rot. Our observations (Y. Luo et al., unpublished data) showed that the area under the spore-density curve (AUSDC) in a dried plum orchard from April 16 to July 31 was 1366, and the incidence of fruit with latent infection at harvest was 38.5%. However, when the AUSDCs in two other orchards were below 200, no fruit with latent infection were detected in either orchard.
|
Fig. 11. Sporulation (conidia) of M. fructicola on thinned dried plum fruit. |
|
|
Fig. 12. Dynamics of spore density of M. fucticola / M. laxa in the air of two dried plum orchards. Solid vertical arrows indicate dates of irrigation in Orchard 1. |
|
Survival of M. fructicola in mummified dried plum fruit in California orchards declines exponentially during the winter (Y. Luo and T. J. Michailides, unpublished data). M. fructicola survived in less than 10% of the mummified fruit 3 months after mummies being partially buried in the ground, while survival was less than 5% of mummies that were hanging on the trees at bloom (Y. Luo et al., unpublished data). Very high humidity in the soil during the wet season in California can accelerate the decaying process of fruit and disintegrate the partially mummified tissues. Furthermore, contamination of mummified fruit by other fungal saprophytes can result in lower survival of Monilinia spp. during the winter. Survival of the pathogen in mummified fruit declines faster at 10 and 15°C (50 and 59°F) than at 5°C (41°F) (Y. Luo et al., unpublished data). Apparently, the mummified fruit bearing the survived pathogen serve as the orchard-based spore inoculum source in spring. The long-distance spore dispersal of M. fructicola and M. laxa conidia and ascospores is still under investigation.
Disease Cycle
The disease cycle of M. fructicola includes two major stages, primary infection of blossoms caused by ascospores or conidia leading to blossom blight in spring, and primary and secondary infection of fruit caused by conidia leading to latent infection and fruit rot in mid- and late-season. Latency of blossom and immature fruit infection is an important feature of the brown rot pathogen. Infections of blossoms can induce blossom blight only when certain factors are present, including favorable microclimatic environmental conditions, blossom susceptibility which depends on the bloom stage, and inoculum potential of the orchard (23). Optimal temperatures for blossom infection and blossom blight expression range from 23 to 25°C (74 to 77°F). The relationship between temperature and proportion of blossom blight fits a one-peak Gaussian function (23). Increasing wetness duration in hours linearly increases incidence of blossom blight. At least 3 to 4 h of wetness are required for blossom infection under the optimal temperature (26), and 1 h of wetness duration may result in a 0.5% increase in blossom incidence (23). The susceptibility of blossoms to infection is moderate at the popcorn stage, maximum at the full bloom stage, and then steadily decreases to the petal fall stage (Figs. 1 and 13A). When blossoms reach the late petal fall stage, the probability of blossom infection and blight can be minimal. Besides the change in susceptibility and despite the fact that the time period from late petal fall to fruit set is too short, infections of blossoms or young fruit continue as latent infections. Higher inoculum concentration can cause significantly higher incidence of blossom blight than lower inoculum concentration does, suggesting that inoculum potential in orchards is a significant determinant in affecting the incidence of blossom blight. The quantitative relationships between incidence of blossom blight and the various factors mentioned above have been described in other studies (23,31).
|
Fig. 13. Incidence of infection of (A) blossoms and (B) latent infection of dried plum by M. fructicola as affected by developmental stage during the season. |
|
When rains occur during bloom of dried plum, conditions for blossom infection are favorable; coinciding with abundant availability of spore inoculum, an incidence of 5 to 10% blossom blight is not uncommon in epidemic years. Fungicide spraying at bloom before the rain event to protect blossoms from infection and blight is a common control strategy followed by the majority of the growers. However, blossom blight is not always a threat, thus fungicide sprays may not be necessary. The bloom period of dried plum (mid March to mid April) is characterized by lower rainfall than that during January and February, and thus the microclimatic conditions are not always favorable for blossom blight to occur. However, surviving blossoms and fruit may still have latent infections which can be very important for the brown rot epidemic. Latently-infected blossoms then may continue to develop and become young fruit bearing latent infections. Furthermore, immature fruit could be infected at all times during the season (if sufficient spore inoculum is available), and conidia produced from mummified fruit on trees or thinned fruit on the ground serve as major sources of inoculum (Fig. 11) for fruit infection during this period. However, fruit infections are normally latent especially in early- and mid-season, but they can lead to fruit rot in late-season when favorable conditions in orchards occur. A large portion of these infected fruit, because they are in clusters and attached to shoots via several fruit stems, do not fall when trees are shaken at harvest. These rotted fruit will dry on the tree, become "mummies", and either can continue hanging on the tree until spring of the following season or drop on to the ground where some of them can produce apothecia and serve as a major source of spore inoculum for the next season.
Fruit susceptibility also changes during the season (Fig. 13B). It is moderate at the fruit set stage, increases to maximum at about the pit hardening stage, and then decreases, reaching the lowest level in early June, at the embryo growth stage. From this point, it then increases again along with fruit development, reaching a moderate level at maturity until harvest (24). This seasonal pattern of fruit susceptibility serves as a basic frame to estimate possible risk of fruit infection at different stages (26).
Major microclimatic factors that affect fruit infection during the season are temperature and wetness duration (24). Similar to the effect on blossom blight, the effect of temperature on fruit infection can be described by a one-peak Gaussian equation with an optimal temperature of fruit infection at about 20 to 21°C (68 to 70°F). However, the effects of temperature on fruit infection can differ at the different fruit developmental stages. Lower temperatures and narrower temperature ranges are required for disease development at early stages than at late stages of fruit development. The incidence of latent infection of fruit increases exponentially with increasing wetness duration in hours, and this relationship remains constant at all developmental stages (24).
The level of latent infection of fruit at different developmental stages correlates significantly with the levels of fruit rot, especially at mid-season after June (26), and a linear equation describes this relationship. This relationship is used to estimate the risk of fruit rot at harvest by detecting latent infection of immature fruit at mid season (see methodology below). However, the probability that a latent infection will lead to fruit rot increases during the season (Fig. 14). In other words, there is a higher chance of a latent infection to develop into a fruit rot in the late season than that in the early season. To determine the risk for fruit rot, one considers both the time when latent infections occur and the microclimatic conditions of orchards in late-season. The total hours of relative humidity greater than 90% (hRH) and total hours of dew period (hDEW) from mid-July to mid-August are critical factors that affect directly (linear correlation) fruit rot. Thus, the threshold conditions that can trigger latent infection to become fruit rot have been determined and include fruit developmental stage, inoculum potential, hRH, and hDEW (26).
|
Fig. 14. Incidences of latent infection and corresponding fruit rot caused by M. fructicola on dried plum during the season. |
|
Methods for Quantifying Latent Infections
Two methods, traditional and molecular, have been developed to detect latent infections. For detecting latent infection by the brown rot fungi in blossoms, the Flower Incubation Technique (FIT) is used. Briefly, this method involves: (i) collection of a sample of 200 to 500 blossoms, depending on the size of the orchard; (ii) surface sterilization of blossoms with 3% commercial bleach for 3 min; (iii) washing with sterile water twice; (iv) incubating at 23 to 25°C (73 to 77°F) for 5 days; and (v) recording blossoms that are covered with sporulation of M. fructicola or M. laxa.
To detect latent infections in immature fruit, the Overnight Freezing-Incubation Technique (ONFIT) is used (36,38). The steps of this technique include: (i) collection of a sample of 100 to 120 immature fruit with the stems attached; (ii) surface sterilization of fruit in a chlorine solution (32 ml of 0.525% sodium hypochlorite, 32 ml 95% ETOH, and 0.01ml surfactant Tween-20 in 2 liters water) for 5 min; (iii) washing the fruit with sterile distilled water twice and placing them in sterile containers; (iv) placing the containers in a freezer at -16°C (3°F) for 10 h and then on a laboratory bench at 23 ± 2°C (73 ± 3.5°F) for 7 days; and (v) recording fruit with evident decay by the brown rot fungi (Fig. 15). Although phenolic acids have been reported to inhibit sporulation and symptom expression of young immature fruit (1), freezing seems to bypass this inhibitory activity and trigger latent infections to form disease symptoms with abundant sporulation produced by the pathogen.
 |
|
 |
Fig. 15. Overnight freezing incubation technique (ONFIT) revealing latent infection expressed as decayed fruit by M. fructicola 5 to 7 days after thawing and incubating at 23 ± 2°C as contrasted to those that after surface sterilization need more than 2 weeks incubation at 23 ± 2°C to develop brown rot symptoms.
Determining blossom infection levels can help estimate, in part, the possible incidence of latently-infected young fruitlets, as well as the risk of fruit rot late in the season. When other means are not available, the incidence of latently-infected blossoms can be used as a parameter to estimate the inoculum potential in orchards for mid- and late-season disease prediction.
Recently, PCR procedures have been developed for the detection of M. fructicola that help to identify the presence or absence of M. fructicola in suspected infected blossoms and in latent infections in various stone fruits, thus providing information on the incidence of infections in a sample. It takes up to 7 days in order to obtain results on the incidence of invisible latent infections by using the ONFIT on immature fruit, or 5 days for directly plating the visible latent infections. Using a PCR technique, multiple samples can be processed and results obtained within 30 hours (37).
Disease Prediction
Three reasons are considered of major importance for the necessity to predict brown rot: (i) Regional-scaled epidemics rarely occur, while orchard-scaled disease development occurs frequently and may depend on several major factors specific to orchards. (ii) Although fungicide applications are still a major disease management strategy, not all orchards require them. (iii) Various situations in individual orchards lead to different disease levels that require different control strategies. Thus, emphasis should be given to prediction of orchard-based disease in the spring for blossom blight and in mid to late season for fruit rot. Estimating the exact disease incidence or severity is still difficult and even unfeasible based on the currently available methodologies. Therefore, disease prediction can provide an estimate of possible risk of disease development.
Risk of blossom blight or fruit latent infection is defined as the probability of occurrence of certain incidence (23). Therefore, the risk can be estimated at different levels. For example, when the probability to have equal or greater than 90% incidence of blossom blight ranges from 0.1 to 0.4, 0.4 to 0.7, or > 0.7, the corresponding risk levels can be arbitrarily designated as low, moderate, and high, respectively (23). Estimation of probability of certain incidence is the core of the risk analysis process, and one useful method is random simulation. This method considers and combines the available mathematical functions or models relevant to disease prediction to estimate an average incidence. The experimental results can be used to analyze the variations contributed by each experimental factor or variable. By using a special computer program, numerous experiments can be simulated, taking into account all possible levels of each experimental factor. The outputs of simulated experiments can be used to produce a distribution of incidence. Any probability of incidence can be estimated from this distribution and the accumulated probability can be used as an estimate of possible risk of a certain incidence. For example, the incidence of blossom blight or fruit rot with 70% accumulated probability means a 70% certainty that the estimated incidence can not exceed a certain incidence value.
A chart of risk of blossom blight takes into account four factors: blossom stage, inoculum potential in the field, temperature, and wetness duration (Fig. 16) (23). Two bloom periods, from popcorn to full bloom and from late full bloom to petal fall, are considered. The inoculum potential is roughly classified into two levels, low and high inoculum levels. Four temperature values are considered, including 10, 15, 20, and 25°C (50, 59, 68, and 77°F). And finally, the wetness duration of 4, 8, 12, 16, 20, and 24 h are considered. This chart provides four risk levels: no risk, low risk, moderate risk, and high risk, based on combinations of different levels of the above four factors. In the majority of the cases when the above factors are considered, the risk levels fall into the "low" to "moderate" categories. Only in a few cases are the "no risk" or "high risk" levels derived from this chart (23).
|
Fig. 16. Chart showing the risks for blossom blight by M. fructicola as affected by bloom developmental stage, inoculum potential, temperature, and wetness duration. |
|
Obviously, an estimation of field inoculum potential is required to accurately predict both blossom and fruit infections. However, it is very difficult to estimate inoculum levels at different fruit developmental stages during the season in orchards. The inoculum potential can be estimated as: (i) the density of mummies on trees bearing sporodochia or mummies on the ground with sporodochia and/or apothecia of the brown rot fungi, (ii) spore density in the air during the season, or (iii) the incidence of rotted fruit in the previous season. The density of thinned fruit bearing sporulation is correlated with the level of fruit latent infection (30), and can be used to roughly estimate the possible risk of fruit rot, although the quantitative relationship between these two variables is still under investigation.
A nested PCR assay (32) has been used to determine efficiently the spore density in the air sampled with spore traps. Two pairs of PCR primers, internal and external, were designed based on the sequence of M. fructicola-specific markers, and the sensitivity of the assay is the DNA of two M. fructicola spores. This assay can detect at least 200 conidia from field samples (i.e., tape of Burkard spore trap). This spore density corresponds to >5% blossom infection under favorable conditions for disease development. This assay is fast, accurate, and very efficient for large samples. However, it still cannot quantify the spore density in the air. Based on the species-specific primers, a real-time PCR assay has been recently developed to quantitatively determine the spore density in the orchard air (Luo et al., unpublished data). This efficient method was used to process all the samples from an entire crop season collected from multiple orchards in different years, and provides a potential methodology for processing large number of samples for use in epidemiological studies.
Another criterion used to estimate inoculum potential in an orchard is the incidence of fruit rot in the previous season. Four levels, <1, 2 to 5, 6 to 10, and > 10% of fruit rot incidence, correspond to very low, low, moderate, and high inoculum potentials, respectively. Combined with the bloom stage and microclimatic environment, this estimate has been used to predict the risk of blossom infection (see below for details).
Fruit infection and fruit rot are dynamic processes during the season. Estimation of risk of latent infection is based on seasonal patterns of fruit susceptibility and inoculum potential in the orchard. In addition, wetness duration is considered as a major environmental factor in the risk analysis process and can be used to estimate possible incidence of latent infection for each fruit developmental stage, making use of the published experimental results (25). Using 1, 5, and 10% as low, moderate, and high incidence of latent infection, respectively, and three corresponding levels of wetness duration at each developmental stage, seasonal patterns (curves) of the "risky" weather can be generated (26). These curves show how the different wetness duration periods at different fruit developmental stages can influence the level of fruit latent infection during the season. Generally, a longer wetness duration period is required at a resistant stage than at a susceptible stage to result in a certain incidence level of latent infection (26). In addition, by using these curves in combination with the information on inoculum potential, an estimation of possible risk of fruit infection can be obtained. For example, historic weather data collected from the California Irrigation Management Information System (CIMIS) for 32 dried plum-growing areas were used to determine the probability of historic occurrence of the "risky" weather, which may induce low, moderate, or high risk of fruit infection determined for 15-day intervals in mid- and late-seasons. The growers can use the frequency of "risky" weather that occurred historically in their orchard location as a reference to estimate the present risk level of fruit infection.
The estimation of latent infection is not the end point of the brown rot disease prediction in season. Different from other stone fruits, which may suffer post-harvest disease problems during cold storage and with the exception of a small amount of dried plum fruit marketed as fresh, the majority of California dried-plums are dehydrated immediately after harvest and marketed as such after further processing. Thus, only the decayed fruit up to harvest result in yield loss while those with latent infection before harvest (no decay symptoms) are dehydrated and considered healthy. Therefore, predicting the probability of fruit bearing latent infections to develop symptoms of disease (decay) is critical in disease management. We did not find a significant correlation between latent infection level of blossoms in spring and fruit rot at harvest based on experiments in a multitude of locations (31). Therefore, blossom infection level cannot be used to predict fruit rot at harvest.
Critical conditions that can trigger latent infection to develop to fruit rot include the incidence of latent infection itself at the corresponding developmental stage (date), inoculum potential, and wetness duration (24) (Fig. 17). In other words, fruit infections that occur at different fruit developmental stages may induce different levels of fruit rot, and certainly different wetness durations may lead to similar levels of fruit latent infection, but result in different levels of fruit rot. Three levels of fruit rot incidence, 1, 5, and 10%, representing low, moderate, and high risk levels, respectively, are assigned (26). The corresponding threshold conditions are used to predict the possible risk of fruit rot before harvest. For instance, an incidence of 11% of latent infection at the late fruit set stage results in a low risk of fruit rot, an incidence of 22.7% at the embryo growth stage results in a moderate risk of fruit rot, while a 37.6% incidence at the late embryo growth stage can result in a high risk of fruit rot (26). The threshold conditions of hRH that induce low, moderate, and high risk of fruit rot are 24, 105, and 204 hours, respectively. The average historic hRHs and hDEWs that induce low, moderate, and high risks of fruit rot, respectively, for 32 locations are also provided as references for disease management.
|
Fig. 17. The incidence of latent infection in relation to fruit growing stage (A), inoculum density (B), and wetness duration (C). |
|
Disease Management
The main principles of integrated brown rot management include: (i) minimizing inoculum potential in orchards, (ii) reducing risk of blossom and fruit infections, and (iii) making correct decisions on disease management strategies. The efficient implementation of all available and effective control strategies becomes a critical factor in disease management.
Fungicides are usually applied at the bloom season to reduce risk of blossom infection and blossom blight in the majority of the California dried plum orchards. The recommended spraying time is at about the green tip stage, but the sprays can continue through white tip and full bloom. Depending upon the weather, the orchard’s disease history, and local environmental conditions, one to three or more fungicide sprays at bloom and on immature fruit may be necessary in mid- and late-season to reduce risk of fruit infection and fruit rot. However, avoiding unnecessary fungicide sprays is an important issue in IPM of brown rot. This information is also updated and posted yearly at the University of California IPM Statewide Program web site.
Several orchard cultural practices are related to changes in inoculum potential. Proper coordination of fruit thinning and irrigation can significantly reduce the inoculum potential. An empirical recommendation is to time the orchard irrigation in such a way so that the thinned fruit on the ground remains for at least 2 weeks without becoming wet. The best time of fruit thinning is from May 10 to May 30, determined by pit-hardening characteristics. Because the end of the rainy season in California usually starts around this period, it is possible to achieve this 2-week dry period unless a rain has occurred. High humidity from a rain, irrigation, or presence of weeds can increase the chance of sporulation produced on Monilinia- infected thinned fruit. However, when the water content of thinned fruit decreases below 10%, only very little sporulation can develop. Weed control can reduce moisture in orchards and also the chance for fruit infection. Removing mummies in the fields may minimize the source of inoculum and reduce disease [shown for peaches and nectarines (15)], however, it is labor and time consuming. Disking the soil can be an effective method in significantly decreasing the density of apothecia and the mummies with sporulation. All orchard sanitation practices can help reduce disease but they may be more costly and labor intensive than the traditional fungicide sprays.
Making the correct decision on timings of fungicide application, fruit thinning, and irrigation are very critical issues in the IPM of brown rot of dried plum. A Decision Support System for IPM of Dried Plum (DSS-PBR) (26,27,28,29) has been developed at the University of California, Kearney Agricultural Center (Fig. 18). Decision support is the main feature of this system, namely, different emphases on disease management are provided at different time periods during the season. Decisions on fungicide sprays at bloom are provided for the March 15 to April 15, and those for the rest of the growing period at 15-day intervals (Table 1). Estimation of risk of blossom infection and timing of fungicide application should be given special emphasis during the bloom period. Three major factors, weather forecast, bloom stage, and estimation of inoculum potential are considered in the blossom blight prediction model, which was developed based on the chart of risks for blossom blight (Fig. 16) (23). Thus, risk level of blossom blight and suggestions on timing of fungicide sprays are provided.
Table 1. Summary of the Decision Support System for IPM of Dried Plum (Prune) Brown Rot (DSS-PBR). Dynamic decisions including fungicide application, fruit thinning, and irrigation are given in different time periods based on input information. A decision model in each time period was developed based on information obtained from experiments reported in the corresponding reference.
Time period |
Required input |
Decision output |
Information used |
Ref. |
 |
 |
 |
 |
 |
 |
 |
 |
 |
 |
 |
 |
 |
3/15 - 4/15 |
|
v |
v |
|
|
v |
|
|
v |
|
v |
|
|
23,30 |
4/16 - 4/30 |
|
|
v |
v |
|
v |
|
|
v |
|
v |
|
|
23,25 |
5/1 - 5/15 |
|
|
v |
v |
|
v |
|
|
v |
v |
v |
v |
|
23,25,26 |
5/16 - 5/31 |
v |
|
|
v |
|
v |
v |
v |
v |
v |
v |
v |
|
23,25,26 |
6/1 - 6/15 |
v |
|
|
|
v |
|
v |
v |
v |
v |
v |
|
v |
23,25,26 |
6/16 - 6/30 |
v |
|
|
|
v |
v |
|
|
v |
v |
v |
|
v |
23,25,26 |
7/1 - 7/15 |
v |
|
|
|
v |
v |
|
|
v |
v |
v |
|
v |
23,25,26 |
7/16 - 7/31 |
v |
|
|
|
v |
v |
|
|
v |
v |
v |
|
v |
23,25,26 |
After bloom, decisions on timing of fungicide sprays to reduce risk of fruit latent infection are based on specific orchard conditions. Decisions during this period are based on fruit developmental stage, inoculum potential, and weather forecast. For orchards with high inoculum potential, risk of inoculum accumulation and fruit infection are also estimated. A suitable period for fruit thinning with recommendations on proper timing of irrigation are also provided. To make decisions on fungicide sprays to reduce the risk of fruit infection several models were used in mid- and late-season. These models have been developed based on experimental published results, including seasonal patterns of fruit susceptibility and risky weather patterns, effects of microclimatic environments on fruit infection, and inoculum dynamics over the season (Table 1). In addition, the threshold conditions triggering latent infection to fruit rot are incorporated into the decision model. This model requires the latent infection level at certain fruit developmental stage in the mid-season as input, calculates the relative probability of latent infection to become fruit rot, and determines the necessity of fungicide spay based on a decision chart (26). A subsequent development of an orchard-based database, using geographic information system (GIS), is in process and will be incorporated eventually into the DSS-PBR in the near future.
Slowing or minimizing fungicide resistance is another important issue in the IPM of brown rot in dried plum. A recent survey of resistance of M. fructicola isolates to three fungicides (51) demonstrated that although none of the tested isolates of M. fructicola were found to be resistant to iprodione, the distribution of EC50 values to iprodione of a current population was significantly different from that of a historic population collected during 1992 to 1998, and the populations have shifted towards to more insensitive isolates. The level of resistance to tebuconazole did not change because this fungicide has been used for only few years in stone fruit orchards in California, and no difference in distribution of EC50 between current and historic populations of isolates was observed. Distribution of EC50 to thiophanate-methyl for the current population of isolates was also significantly different from that of historic (1998) isolates. Three groups having distinct ranges of EC50 values to thiophanate methyl were found, benzimidazole-sensitive, low-resistant, and high-resistant isolates. These three groups were equally pathogenic under non-fungicide treatment. However, the high-resistant isolates caused significantly higher incidence of blossom blight than the low-resistant isolates after a thiophanate methyl treatment, and the frequency of low-resistant isolates was significantly reduced. The results of the above survey indicated that a heavy use of fungicides can select resistance in the pathogen population. However, using fungicides with different modes of action may prolong the effectiveness of fungicides in disease control and slow fungicide resistance.
Monitoring fungicide resistance in pathogen populations has become an important process in alternative fungicide applications in disease management. Efficient quantification of fungicide-resistant genotypes in a population will greatly help in making decisions on alternative fungicide sprays to reduce risk of development of fungicide resistance. We (Luo et al., unpublished data) recently developed a real-time PCR assay to quantify the allele E198A which confers high resistance of M. fructicola isolate population to benzimidazole. This newly-developed and efficient method provides a potential tool for efficient surveys on fungicide resistance levels at both field and regional scales.
Summary
The various developments completed from studies on dried plums can have application on other stone fruit, such as peaches, nectarines, plums, apricots, and cherries grown in California. A similar seasonal pattern of fruit susceptibility to M. fructicola was also reported on peach and nectarine fruit (18) and detached peach fruit (3). Thus, at least the trend of change in susceptibility of stone fruits during the season might be similar to that in dried plum. The conclusion on the sporulation of Monilinia spp. on thinned fruit serving as source of inoculum in mid-season in dried plum orchards was similar to that on peaches (22) and to that determined with thinned fruit of nectarines (16). Temperature effects on infection of peach and sweet cherry fruit (5) and on sporulation of M. fructicola (44) are similar to those determined for dried plums and can be used in future studies of other stone fruits. Quantitative effects of wetness duration on infection of peach fruit (5) and cherry (21) by the pathogen reported previously were also similar to those on dried plums. The quantitative effects of inoculum concentration on blossom blight (50) and detached fruit (42) of cherry were confirmed. These effects were also detected on blossoms of peaches and nectarines recently (Luo et al, unpublished data). Therefore, the chart of risk of blossom blight for dried plum (23) can be generally applied for brown rot of other stone fruits. For example, our blossom blight risk model was tested using multi-year data of blossom blight of cherries and peaches in Oregon (Jay W. Pscheidt, Personal communication), and the results demonstrated that the model is applicable in predicting the brown rot risk under conditions of Oregon. Since latent infection of blossoms and fruit are commonly encountered in other stone fruit, such as peaches (11,20), plums (43), cherries (1), and apricots (10,49), the principles of risk analysis for blossom blight in spring and latent infection in mid- and late-season may have applications in these stone fruit also. However, for more accurate predictions, detailed studies as those completed on dried plum should be done for the other stone fruits.
The exponential decline of surviving M. fructicola in mummies over the winter should be confirmed using mummies from other stone fruit. The percentage of mummies with sporulation that remained on trees in the spring in a plum orchard was found to be significantly higher than in a dried plum orchard (Luo et al., unpublished data). Understandably, the length of time needed to completely dry and become a mummy increases as the size of mummified fruit increases. Because peach, nectarine, and plum fruit are generally larger than dried plums, there is a higher chance for more completely mummified fruit with viable M. fructicola in dried plum orchards than in orchards of other stone fruit. Studies to determine the quantitative relationships between microclimatic conditions and inoculum potentials and level of latent infection of peaches and nectarines are in progress. The results may help understand differences in brown rot development in mid-season between dried plum and other stone fruits. Since all other stone fruit may have post-harvest problems in cold storage, thresholds for disease management should be different from and stricter than those established for dried plums. Whether the conditions that trigger latent infection for fruit rot development in other stone fruits are similar to those for dried plum is still unknown. In addition, the conclusion about the importance of latent infection during the different fruit developmental stages in contributing to fruit rot for dried plum still needs to be confirmed for the other stone fruits.
Decision support for fungicide sprays to manage fungicide resistance is a critical issue of the DSS-PBR. Orchard- and region-scaled surveys for fungicide resistance are needed to understand the current situation of fungicide resistance and the geographic distribution of resistant pathogen populations. Prediction of fungicide resistance development needs a modeling approach which incorporates relevant parameters obtained experimentally. Such a model could also serve as a tool to evaluate the results of various grower strategies to manage resistance in the brown rot fungi of stone fruit in California. The best strategies could be then selected based on an optimization approach.
To improve the DSS-PBR developed for dried plum one needs to consider several issues. All modules of the system need to be tested continuously, evaluated, and modified by using multi-year and multi-location experimental data. Simplifying the use of the system is a continuous task in our laboratory, with major efforts devoted towards an accurate quantification of inoculum potential in orchards and a user-friendly retrieval of the weather forecast by the growers. Completion of the orchard-based databases can provide regional disease predictions and better implementation of disease management approaches. An economic analysis can be another very useful tool that will help growers make decisions on disease management. An improved decision system will save growers unnecessary costs, protect the environment from unnecessary sprays, and provide more efficient brown rot management in California stone fruit.
Future Prospects
Our near future goal is to develop a decision support system for IPM of brown rot of all stone fruits grown in California. By extending the information developed in the DSS-PRB to other stone fruits, after obtaining new experimental information from the other stone fruits, the system could be developed in such a way so that it is specific for peach, nectarine, plum, apricot, and cherry. Because peach, nectarine, plum, and cherry cultivation include many commercial varieties, the effects and differences among all these varieties on disease development could be of major importance and should be also considered. A far future goal of our research is the establishment of a computerized program that any California stone fruit growers can use to obtain help in making decisions for brown rot management in their orchards.
Literature Cited
1. Adaskaveg, J. E., Förster, H., and Thompson, D. F. 2000. Identification and etiology of visible quiescent infections of Monilinia fructicola and Botrytis cinerea in sweet cherry fruit. Plant Dis. 84:328-333.
2. Bostock, R. M., Wilcox, S. M., Wang, G., and Adaskaveg, J. E. 1999. Suppression of Monilinia fructicola cutinase production by peach fruit surface phenolic acids. Physiol. Molec. Plant Pathol. 54:37-50.
3. Biggs, A. R., and Northover, J. 1985. Inoculum sources for Monilinia fructicola in Ontario peach orchards. Can. J. Plant Pathol. 7:302-307.
4. Biggs, A. R., and Northover, J. 1988. Early and late-season susceptibility of peach fruits to Monilinia fructicola. Plant Dis. 72:1070-1074.
5. Biggs, A. R., and Northover, J. 1988. Influence of temperature and wetness duration on infection of peach and sweet cherry fruits by Monilinia fructicola. Phytopathology 78:1352-1356.
6. Boehm, E. W. A., Ma, Z., and Michailides, T. J. 2001. Species-specific detection of Monilinia fructicola from California stone fruits and flowers. Phytopathology 91:428-439.
7. Burt, A., Carter, D. A., Koenig, G. L., White, T. J., and Taylor, J. W. 1996. Molecular markers reveal cryptic sex in the human pathogen Coccidioides immitis. Proc. Natl. Acad. Sci. USA 93:770-773.
8. Byrde, R. J. W., and Willetts, H. J. 1977. The Brown Rot Fungi of Fruit: Their Biology and Control. Pergamon Press. Oxford and New York.
9. Corbin, J. B. 1963. Factors determining the length of the incubation period of Monilinia fructicola (Wint.) Honey in fruits of Prunus spp. Aust. J. Agric. Res. 14:51-60.
10. Cruickshank, R. H., and Wade, G. C. 1992. The activation of latent infections of Monilinia fructicola on apricots by volatiles from the ripening fruit. J. Phytopath. 136:107-112.
11. Emery, K. M., Michailides, T. J., and Scherm, H. 2000. Incidence of latent infection of immature peach fruit by Monilinia fructicola and relationship to brown rot in Georgia. Plant Dis. 84:853-857.
12. Förster, H., and Adaskaveg, J. E. 2000. Early brown rot infections in sweet cherry fruit are detected by Monilinia-specific DNA primers. Phytopathology 90:171-178.
13. Fulton, C. E., and Brown, A. E. 1997. Use of SSU rDNA group-I intron to distinguish Monilinia fructicola from M. laxa and M. fructigena. FEMS Microbiol. Lett. 157:307-312.
14. Fulton, C. E., van Leeuwen, G. C. M., and Brown, A. E. 1999. Genetic variation among and within Monilinia species causing brown rot of stone and pone fruits. Euro. J. Plant Pathol. 105:495-500.
15. Holtz, B. A., Michailides, T. J., and Hong, C. X. 1998. Development of apothecia from stone fruit infected and stromatized by Monilinia fructicola in California. Plant Dis. 82:1375-1380.
16. Hong, C. X., Holtz, B. A., Morgan, D. P., and Michailides, T. J. 1997. Significance of thinned fruit as a source of the secondary inoculum of Monilinia fructicola in California nectarine orchards. Plant Dis. 81:519-524.
17. Hong, C, X., and Michailides, T. J. 1998. Effect of temperature on the discharge and germination of ascospores by apothecia of Monilinia fructicola. Plant Dis. 82:195-202.
18. Ibbotson-Darhower, H., Hickey, K. D., and Travis, J. W. 1998. Susceptibility of peach and nectarine fruit at different developmental stages to Monilinia fructicola. (Abstr.) Phytopathology 88:S130 (Suppl.).
19. Ioos, R., and Frey, P. 2000. Genomic variation within Monilinia laxa, M. fructigena and M. fructicola and application to species identification by PCR. Euro. J. Plant Pathol. 106:373-378.
20. Jerome, S. M. R. 1958. Brown rot of stone fruits: Latent contamination in relation to spread of the disease. J. Aust. Inst. Agric. Sci. 24:132-140.
21. Koball, D. C., Wilcox, W. F., and Seem, R. C. 1997. Influence of incubation-period humidity on the development of brown rot blossom blight of sour cherry. Phytopathology 87:42-49.
22. Landgraf, F. A., and Zehr, E. 1982. Inoculum sources for Monilinia fructicola in South Carolina peach orchards. Phytopathology 72:185-190.
23. Luo, Y., Morgan, D. P., and Michailides, T. J. 2001. Risk analysis of brown rot blossom blight of prune caused by Monilinia fructicola. Phytopathology 91:759-768.
24. Luo, Y., and Michailides, T. J. 2001. Factors affecting latent infection of prune fruit by Monilinia fructicola. Phytopathology 91:864-872.
25. Luo, Y., Ma, Z., and Michailides, T. J. 2001. Analysis of factors affecting latent infection and sporulation of Monilinia fructicola on prune fruit. Plant Dis. 85:999-1003.
26. Luo, Y., and Michailides, T. J. 2001. Risk analysis for latent infection of prune by Monilinia fructicola in California. Phytopathology 91:1197-1208.
27. Luo, Y., and Michailides, T. J. 2003. Threshold conditions that lead latent infection to prune fruit rot caused by Monilinia fructicola. Phytopathology 93:102-111.
28. Luo, Y., Michailides, T. J. 2001. Development of website for brown rot of stone fruits and a decision support system for IPM of blossom blight of dried plum. Pages 53-59 in: 2001 Prune Res. Rep. and Index of Prune Res., California Prune Board, Pleasanton, CA.
29. Luo, Y., Michailides, T. J. 2002. Improvement of the decision support system for IPM of prune brown rot (DSS-PBR) in 2002. Pages 68-70 in: 2002 Prune Res. Rep. and Index of Prune Res., California Prune Board, Pleasanton, CA.
30. Luo, Y., Michailides, T. J., Ma, Zhonghua, Morgan, D. P., W. H. Krueger, and R. P. Buchner. 2002. Prediction of brown rot of dried plum: spore inoculum potential in orchards and best time period for determining fruit latent infection caused by Monilinia fructicola. Pages 55-67 in: 2002 Prune Res. Rep. and Index of Prune Res., California Prune Board, Pleasanton, CA.
31. Luo, Y., Michailides, T. J., Morgan, P. D., Krueger, W. H., and Buchner, R. P. 2005. Inoculum dynamics, fruit infection, and development of brown rot in prune orchards in California. Phytopathology 95:1132-1136.
32. Ma, Z., Luo, Y., and Michailides, T. J. 2003. Nested PCR assays for detection of Monilinia fructicola in stone fruit orchards and Botryosphaeria dothidea from pistachios in California. J. Phytopath. 151:312-322.
33. Michailides, T. J. 1991. Russeting and russet scab of prune, an environmentally induced fruit disorder: Symptomatology, induction, and control. Plant Dis. 75:1114-1123.
34. Michailides, T. J., and Morgan, D. P. 1993. Wind scab of French prune: Symptomatology and predisposition to preharvest and postharvest fungal decay. Plant Dis. 77:90-95.
35. Michailides, T. J., and Morgan, D. P. 1997. Influence of fruit-to-fruit contact on the susceptibility of French prune to infection by Monilinia fructicola. Plant Dis. 81:1416-1424.
36. Michailides, T. J., Morgan, D. P., and Felts, D. 2000. Detection and significance of symptomless latent infection of Monilinia fructicola in California stone fruit. (Abstr.) Phytopathology 90:S48.
37. Michailides, T. J., D. P. Morgan, Z. Ma, Y. Luo, D. Felts, M. A. Doster, and H. Reyes. 2005. Conventional and molecular assays aid diagnosis of crop diseases and fungicide resistance. California Agriculture 59:115-123.
38. Michailides, T. J., Morgan, D. P., Felts, D., and Krueger, W. 1996. Ecology and epidemiology of prune brown rot and new control strategies. Pages 109-123 in: 1996 Prune Res. Rep. and Index of Prune Res., California Prune Board, Pleasanton, CA.
39. Michailides, T. J., Ogawa, J. M., and Opgenorth, D. C. 1987. Shift of Monilinia spp. and distribution of isolates sensitive and resistant to benomyl in California prune and apricot orchards. Plant Dis. 71:893-896.
40. Michailides, T. J., Ogawa, J. M., and Sholberg, P. L. 1987. Chemical control of fungi causing decay of fresh prunes during storage. Plant Dis. 71:14-17.
41. Nei, M. 1973. Analysis of gene diversity in subdivided populations. Proc. Natl. Acad. Sci. USA 70:3321-3323.
42. Northover, J., and Biggs, A. R. 1995. Effect of conidial concentration of Monilinia fructicola on brown rot development in detached cherries. Can. J. Plant Pathol. 17:205-214.
43. Northover, J., and Cerkauskas, R. F. 1994. Detection and significance of symptomless latent infections of Monilinia fructicola in plums. Can. J. Plant Pathol. 16:30-36.
44. Phillips, D. J. 1984. Effect of temperature on Monilinia fructicola spores produced on fresh stone fruits. Plant Dis. 68:610-612.
45. Polito, V. S. 1981. Flower and fruit development. Pages 46-52 in: Prune Orchard Management. D. E. Ramos, ed. Division of Agricultural Sciences, University of California. Berkeley, CA.
46. Sholberg, P. L., Ogawa, J. M., and Manji, B. T. 1981. Diseases of Prune blossoms, fruits, and leaves. Pages 121-125 in: Prune Orchard Management. D. E. Ramos, ed. Division of Agricultural Sciences, University of California, Berkeley, CA.
47. Sinclair, J. B, and Cerkauskas, R. F. 1996. Latent infection vs. endophytic colonization by fungi. Pages 3-29 in: Endophytic Fungi in Grasses and Woody Plants, S. C. Redlin and L. M. Carris, eds. APS Press, St. Paul, MN.
48. Taylor, J. W., Geiser, D. M., Burt, A., and Koufopanou, V. 1999. The evolutionary biology and population genetics underlying fungal strain typing. Clin. Microbiol. Rev. 12:126-146.
49. Wade, G. C., and Cruickshank, R. H. 1992. The establishment and structure of latent infections with Monilinia fructicola on apricot. J. Phytopath. 136:95-106.
50. Wilcox. W. F. 1989. Influence of environment and inoculum density on the incidence of brown rot blossom blight of sour cherry. Phytopathology 79:530-534.
51. Yoshimura, M. A., Luo, Y., Ma, Z., and Michailides, T. J. 2004. Sensitivity of Monilinia fructicola from stone fruit to thiophanate-methyl, iprodione, and tebuconazole. Plant Dis. 88:373-378.