Common Bean and Its Importance for the Brazilian Population
Brazil is among the largest growers and consumers of common beans (Phaseolus vulgaris). They are a well-known source of protein, carbohydrates, and micronutrients for millions of people worldwide (http://www.cgiar.org/our-strategy/crop-factsheets/beans/). Beans are also a good source of calories. Along with their good nutritional qualities, their long storage life and relative ease of preparation make them one of the most important diet components in many developing countries. This is particularly true for families dependent on agriculture for their living, notably in Africa and in Latin America (Broughton et al. 2003).
In Brazil, common beans are very popular and represent a major source of dietary protein. The Brazilian per capita consumption is around 17 kg/year. The total growing area in 2015 was 1.94 million ha, with a mean yield of 1,390 kg/ha (Feijão 2016). In the period between 2002 and 2015, the total production in Brazil varied between 2.9 and 3.7 million tons yearly. These totals still may not supply the demand, with yearly imports typically ranging from nearly 100,000 tons to more than 300,000 tons.
 |
Fig. 1. Electron micrograph of partially purified Bean golden mosaic virus particles. |
Typically, Brazil has three growing seasons for common beans. The “first growing season” is also called the “rainy season,” when beans are sown from August through November (and represents an average of 49.4% of the total production); the “second growing season” or “dry season” usually goes from mid-February to mid-March (representing an average of 45.3% of the total production); and during the “third growing season,” or “irrigated crop” or “winter crop,” bean is sown between April and July (representing an average of 5.3% of the total production). This third planting season is possible in part of the Central-West and Southeast regions of the country (Feijão 2016).
Disease, insect pests, drought, and low soil fertility are among the most important yield constraints, particularly in tropical regions, that prevent the expression of the cultivar’s full yield potential. Together, these constraints may cause production instability, thus also affecting food security. The Brazilian states of Paraná, Minas Gerais, Bahia, São Paulo, and Goiás are the five main growers. Since the 1970s, the incidence of Bean golden mosaic virus (BGMV) has made it probably the most devastating viral disease of common beans in these states. The effect of this disease alone may account for the importation needs of the country.
Golden Mosaic of Common Beans (Bean golden mosaic virus)
 |
Fig. 2. (A) Nuclear inclusion body and nucleolar hypertrophy seen by light microscopy in bean leaf infected by Bean golden mosaic virus. I = inclusion body, n = nucleolus. Bar 10 µm. (B) Fibrilar ring observed by electron microscopy in the nucleus of bean leaf infected by Bean golden mosaic virus. Fr = fibrilar ring, n = nucleus. Bar = 1µm. Reproduced from Gilbertson et al. 1991. Plant Dis. 75:336-342. (C) Immunogold labeled aggregate of BGMV particles in the nucleus of an infected bean leaf phloem companion cell, next to the nucleolus. Bar = 0.5 mm. |
While the science of geminivirology can be traced back to Baur (1905) [cited by Morales and Andersen (2001)], the discovery of what would be named geminiviruses was first described by a group of plant virologists from Brazil (Costa 1965). Costa (1937) investigated the mosaic disease of the cultivated malvaceous plant cotton (Gossypium hirsutum). Silberschmidt (1943) published the Studies on the Experimental Transmission of the Infectious Chlorosis of the Malvaceae, indicating his interest in the studies conducted by Baur on the etiology of malvaceous chlorosis. Orlando and Silberschmidt (1946) first observed that the causal agent of the “infectious chlorosis of Malvaceae” was whitefly-transmitted (by Bemisia tabaci (Morales and Andersen 2001). The geminiviruses belong to the Geminiviridae family and infect a wide range of economically important crop species such as common bean, soybean (Glycine max), cassava (Manihot esculenta), tomato (Solanum lycopersicum), maize (Zea mays), cotton (Gossypium hirsutum), and wheat (Triticum aestivum). They have been recognized as a major threat to agriculture worldwide.
Bean golden mosaic virus (BGMV) is a single-stranded DNA virus (family Geminiviridae, genus Begomovirus) whose genome has two components of about 2.6 kb in length each, typical of the New World geminiviruses (Gilbertson et al. 1993), both of which are necessary for plant infectivity. These molecules are packed into geminated particles about 17 nm in diameter, unique for the viral world (Fig. 1). Nuclear inclusions in the phloem cells, typical for geminiviruses, can be seen using light microscopy, along with hypertrophy of nuclei and nucleolar elongation. The unique presence of fibrillar rings in the nuclei of phloem parenchyma cells can be seen by electron microscopy (Fig. 2).
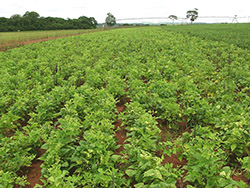 |
Fig. 3. Bean field of cv. Jalo Precoce with typical symptoms of Bean golden mosaic virus on aerial parts. |
The main symptoms characteristic of the disease are the yellow-green mosaic of leaves (Figs. 3 and 4), variable levels of curling, generally stunted growth, and distorted pods (Fig. 5), which may vary among the cultivated genotypes and time of infection and may also include the loss of apical dominance (Fig. 6) in many situations (witches’-broom symptoms) (Figs. 7, 8, and 9). While this virus is essentially restricted to common beans and other Phaseolus species such as lima beans (P. lunatus), it has also been reported naturally occurring in soybean (Fernandes et al. 2009) and wild bush bean or quail bean (Macroptillium lathyroides) (Lima et al. 2013), a synonymous species of Phaseolus lathyroides. The disease was initially described in 1961 by Costa (Costa 1965) as one of limited importance. However, by 1975, Costa had observed extremely high populations of B. tabaci associated with the ever-expanding soybean crop in the Brazilian states of Paraná and São Paulo, and warned of the threat to the bean crop due to bean golden mosaic. With the introduction of the B biotype of B. tabaci in the early 1990s in Brazil (Lourenção and Nagai 1994), another significant increase in the whitefly population was observed in several crops, including soybean. By this time soybean was already known to be an excellent host for BGMV, even though it does not seem to be important for this crop. As the soybean continued to expand, so did the whitefly population and, as a result, BGMV took over the states of Parana, São Paulo, Goiás, Minas Gerais, and Bahia. Soybean is believed to be the main cultivated BGMV host plant. In fact, BGMV became widespread wherever common bean is grown except in the extreme south of Brazil. The dry bean season crop has been reduced, owing mostly to this disease. This reduction in production is being replaced in Central-West Brazil by the winter crop. This production is using higher inputs yet being rewarded with higher yields due to a decrease in whitefly populations after soybean harvest. BGMV alone may account for up to 100% yield losses in a single field in many bean-growing areas of Brazil, depending upon time of infection. Annual average yield losses have been estimated at 20%. It is worth mentioning that BGMV is not as easily transmissible mechanically as its Central American counterpart, Bean golden yellow mosaic virus (BGYMV) (Costa 1976; Garrido-Ramirez et al. 2000).
Unlike many other begomoviruses, Faria and Maxwell (1999) found low levels of genetic variability in BGMV. Many partial and complete BGMV sequences were deposited in the National Center for Biotechnology Information’s GenBank since the first complete clones were deposited in 1989 (Gilbertson et al. 1993). Recently, Ramos Sobrinho et al. (2014) confirmed the low genetic diversity of BGMV after examining full-length clones of the virus from various regions of Brazil.
 |
 |
 |
Fig. 4. Symptoms of golden mosaic on bean plants under field situation. |
Fig. 5. Detail of cv. Jalo Precoce showing severe pod damage by Bean golden mosaic virus. |
Fig. 6. Bean line DOR 303 showing severe loss of apical dominance after infection by Bean golden mosaic virus under greenhouse conditions. |
The BGMV Whitefly Vector: Bemisia tabaci
BGMV is transmitted in a persistent, non-propagative manner by the vector Bemisia tabaci (Hemiptera: Aleyrodidae). Early experiments conducted by Costa (1976) indicated that the insect acquires the virus after 10 to 15 min and can retain transmissibility for up to 21 days. It is believed that persistent, circulative transmission does occur for some geminiviruses; however, replication in the vector is questioned (Rosen et al. 2015).
Bemisia tabaci is a complex group of cryptic species—referred to as haplotypes, usually called biotypes—some of which are well characterized (de Barro et al. 2011; Dinsdale et al. 2010; Gill and Brown 2010). Bemisia tabaci stands out among more than 1000 species of whiteflies due to its adaptability, persistence, and potential to damage several crop species (Stansly and Naranjo 2010). It is a phloem-feeding insect pest and plant virus vector of agricultural crops and uncultivated eudicots in tropical and subtropical regions worldwide. Damage to the host plant may be caused by direct feeding and deposition of honeydew (the excreted waste product) on which sooty mold fungi can grow and reduce photosynthesis (Oliveira et al. 2001). In addition, B. tabaci is the vector of several plant virus genera, of which the most widespread and damaging are the begomoviruses (Brown et al. 2015).
There are at least four biotypes of B. tabaci currently reported in Brazil, based on analysis of the mitochondrial cytochrome oxidase I gene (mtCOI) (de Barro et al. 2011; Dinsdale et al. 2010), including B. tabaci MEAM1 (Middle East-Asia Minor 1) (Biotype B), B. tabaci NW (New World) (Biotype A), B. tabaci NW2 (New World 2), and B. tabaci MED (Mediterranean; Biotype Q) (Barbosa et al. 2015). Biotype B has recently been reported to complete its developmental cycle on maize plants, both under field and under greenhouse conditions (Quintela et al. 2016). This expands the host range of the B biotype to monocot hosts, thus providing another source of increased infestation, which can eventually migrate to common beans.
 |
 |
Fig. 7. Symptom of loss of apical dominance (witches’-broom) which may be the result of multiple virus infections under field conditions. |
Fig. 8. Symptoms of mixed infection caused by Bean golden mosaic virus and Cowpea mild mottle virus on common bean under field conditions.
|
Breeding for BGMV Resistant Varieties
Breeding for golden mosaic resistance started soon after recognition of its importance in 1970 in the State of São Paulo. Tulmann et al. (1977) used gamma radiation and ethyl methane sulfonate (EMS) in thousands of seeds of commercial varieties to attempt to create variability for disease resistance. Unfortunately, no single commercial cultivar derived from those efforts was released; however, the varietyTMD-1, was identified and used as source of resistance by Bianchini (1999).
By 1977, bean lines considered to possess field-resistance to BGMV such as Aeté1/37, Aeté 1/38 and Aeté1/40 were found to have been escapes rather than resistant reactions after early infection was evaluated in other trials. In the 1980s Embrapa Arroz e Feijão released the cultivar Onix, a black bean with moderate tolerance to the disease; however, it had no real impact, mostly owing to its type of plant architecture. The Instituto Agronômico do Paraná (IAPAR) developed several cultivars such as IAPAR 57, IAPAR 65, IAPAR 72 and more recently IPR Eldorado. Unfortunately, they all failed under moderate or severe epidemics of BGMV (J. C. Faria, personal observation). A recurrent selection program for tolerance to BGMV was initiated at Embrapa Arroz e Feijão in the 1990s. The starting germplasm was able to delay symptoms, with less severe yellowing and stunting than most accessions. In spite of the efforts by several researchers, after 25 years the program could not yet release a highly resistant commercial common bean cultivar with acceptable disease tolerance. Inheritance studies conducted independently have indicated that disease tolerance is a complex trait for which to select. Pessoni et al. (1997) indicated that plant dwarfing, foliar yellowing, and pod malformation characteristics, using a weighted generation means analysis, could be selected for at the same time. The additive gene action component was significant, and there was a positive correlation among the three traits.
Because of the difficulties in breeding for disease resistance using traditional techniques, the main measure taken to manage the disease has been the chemical control of the whitefly vector B. tabaci. Chemical control may not be effective due to the whitefly’s efficient transmission ability and high populations created with other crops grown in the vicinity of bean fields. An effective control mechanism continues to elude both plant breeders and plant pathologists as well as entomologists because no natural immunity or high level of disease resistance has so far been identified in any genotypes of Phaseolus (Faria et al. 2006; Morales and Anderson 2001). The transgenic approach to managing the disease is a very important component, but managing the whitefly population continues to be important because of other whitefly-borne viruses as well as direct insect damage.
The Development of a BGMV-Resistant Common Bean Transgenic Event
 |
Fig. 9. Partial view of a high-tech farmer field completely taken by Bean golden mosaic virus infection. |
In 1986, the pioneering work of Roger Beachy´s group demonstrated that the concept of transgenic pathogen-derived resistance (PDR) utilizing coat protein-mediated resistance to TMV was feasible (Abel et al. 1986; Beachy 1999). This approach was also applied to the development of the genetically modified papaya resistant to Papaya ringspot virus, which has been commercially cultivated in Hawaii since 1998 (Gonsalves et al. 2004). Based on these examples, work began in the early 1990s on the development of a common bean line immune to BGMV. We molecularly characterized the biology and diversity among BGMV isolates occurring in Brazil, allowing us to construct the first genetic engineering strategies.
In addition, we had to develop systems for genetic transformation of P. vulgaris, which is still one of the most difficult species to manipulate via genetic engineering. We have achieved an efficient transformation system that allowed us to obtain the first transgenic lines in 1993 (Aragão and Rech 2001; Aragão et al. 2008). This system generated a transgenic line engineered to express the BGYMV coat protein gene—the best option for PDR—but it failed to show resistance to the virus (D. P. Maxwell, unpublished data). The next step was to explore the expression of viral genes (rep, TrAP, and ren) in the antisense orientation. The mechanism of RNA interference (RNAi) and its biological functions were not understood at that time. The obtained transgenic products resulted in delayed and attenuated golden mosaic symptoms upon whitefly-mediated inoculation (Aragão et al. 1998).
 |
Fig. 10. Bean golden mosaic virus susceptible control plot and border rows from a 2013 field experiment assaying transgenic common bean using the RNAi strategy. The control common bean is displaying severe and uniform infection symptoms, while the transgenic plot (front) is free of Bean golden mosaic virus symptoms. |
The technique of lethal transdominance was later used to generate plants expressing the BGMV mutated rep gene, which encodes the only protein essential for viral genome replication. One of the lines (named M1/4) exhibited resistance to the virus. However, it was demonstrated that the resistance was dependent on the number of viruliferous whiteflies utilized at inoculation (Faria et al. 2006). This line was tested under field conditions, but this strategy was discontinued after finding that incidence of BGMV infection increased with increased viruliferous whitefly populations, reaching levels that were unacceptable for a GM technology.
Pursuing our initial objective of generating a bean line with immunity to BGMV, we decided to explore the concept of RNAi, then not yet well understood, to silence the rep viral gene (Bonfim et al. 2007). We hypothesized that silencing the expression of this gene would prevent viral DNA replication and, consequently, the appearance of symptoms. Among the GM lines generated with an intron-hairpin construct designed to induce silencing of the rep gene, two candidates displayed an immune response after inoculation with hundreds of viruliferous whiteflies per plant. Homozygous plants showed 100% immunity under field conditions, while non-transgenic plants showed severe symptoms characteristic of golden mosaic disease (Aragão and Faria 2009). This immunity towards BGMV infection under field conditions continues to be maintained since 2007.
Off-target or epigenetic effects were not apparent, and transgenic lines always showed normal phenotypes. We selected the transgenic line 5.1 for biosafety analyses that were carried out by the Biosafety Network of Embrapa. All risk assessment data demonstrated no differences between transgenic lines and parental plants; therefore, we concluded that the line 5.1 is safe for the environment and human consumption (Aragão and Faria 2010; Aragão et al. 2013; Carvalho et al. 2015; Faria et al. 2014; Paula et al. 2015). Based on these data, in 2011 the Brazilian Biosafety Committee (CTNBio) approved this line for cultivation and human consumption in Brazil (http://ctnbio.mcti.gov.br/liberacao-comercial#/liberacao-comercial/consultar-processo).
When CTNBio approved the line 5.1, advanced transgenic breeding lines with “Carioca” seed type had already been developed. Although not grown in other parts of the world, “Carioca” beans are the most-consumed class of market grain in Brazil, representing around 70% of the national market. Field trials to evaluate the agronomic performance of the transgenic BGMV-resistant lines, which are required for the registration of new cultivars in Brazil, started in 2012 in a specifically designed National Field Trial Network coordinated by Embrapa (Figs. 10, 11, and 12). The seed production process (breeder and pre-basic seeds) also began in 2012 (Fig. 13). Results from 31 such field trials demonstrated that no grain yield or commercial grain quality penalties have been observed when the transgenic lines were compared with their respective recurrent parents (Table 1). The transgenic lines maintained their resistance to BGMV across all tested field conditions since then. Disease incidence levels ranging from 50 to 70% in the conventional recurrent parents and other control cultivars were documented occurring in eight environments with high natural golden mosaic infection (Fig.14). There were additional trials where 100% BGMV incidence and severity was observed in the conventional control cultivars not included in the 31 trials mentioned above. The transgenic lines from these additional trials remained immune without exhibiting any golden mosaic symptoms.
 |
 |
Fig. 11. Advanced bean field plot, 2016: (left) transgenic RNAi common bean displaying completely resistant to Bean golden mosaic virus; (right) control plot (non-GM) with uniform infection by Bean golden mosaic virus. |
Fig. 12. Advanced bean field plot, 2016: (left) control plot (non-GM) with uniform infection by Bean golden mosaic virus; (right) transgenic RNAi common bean displaying complete resistance to Bean golden mosaic virus. |
 |
 |
Fig. 13. Genetic seed production field of transgenic bean line CNFCT16207. |
Fig. 14. Bean golden mosaic virus severity in transgenic common bean advanced lines and conventional control cultivars in eight environments in Brazil. Severity was estimated using a standard scoring scale ranging from 1 to 9, where: 1 = 0.0% of the experimental unit affected by disease; 2 = up to 1.0%; 3 = 1.1 to 5.0%; 4 = 5.1 to 10.0%; 5 = 10.1 to 20.0%; 6 = 20.1 to 40.0%; 7 = 40.1 to 60.0%; 8 = 60.1 to 80.0%; and 9 = 80.1 to 100.0%. |

On the other hand, all tested common bean lines (transgenic and non-transgenic) were susceptible to Cowpea mild mottle virus (CPMMV). However, accurate evaluation of CPMMV severity was accomplished only in the transgenic line plots, because the CPMMV symptoms were completely masked by the golden mosaic symptoms in the conventional cultivar plots. Disease severity ranging from 17 to 69% was observed in five trials with high natural infection of CPMMV (Fig. 15).
 |
Fig. 15. Severity of Cowpea mild mottle virus in transgenic common bean advanced lines in five environments in Brazil. Severity was estimated using a standard scoring scale ranging from 1 to 9, where: 1 = 0.0% of the experimental unit affected by disease; 2 = up to 1.0%; 3 = 1.1 to 5.0%; 4 = 5.1 to 10.0%; 5 = 10.1 to 20.0%; 6 = 20.1 to 40.0%; 7 = 40.1 to 60.0%; 8 = 60.1 to 80.0%; and 9 = 80.1 to 100.0%. |
A transgenic common bean line with superior agronomic performance in the field trials was selected and registered as cultivar BRS FC401 RMD, becoming the first transgenic common bean cultivar in the world. When compared to the control recurrent parents BRS Pontal and Pérola, this transgenic BGMV-resistant cultivar had a grain yield increase over the two conventional cultivars of 13.6%, considering all 31 tested environments. Considering 14 trials conducted during the second growing season, or “dry season”—the main target for the transgenic bean due to the high level of whitefly population in the field—the grain yield superiority was 42.6%. Of the 14 environments above, considering only the four with the highest BGMV incidence and severity, the grain yield increase over the two conventional cultivars reached 174.3% (Table 2).
GM technologies for the control of viruses should be used in a context of good pest control practices directed by the principles of integrated pest management, which have to take into consideration the integration of all available pest control methods that are socially and economically acceptable. Although immunity to BGMV has been demonstrated through the use of the genetically modified bean under field conditions since 2007, other whitefly-transmitted viruses must be addressed. Consequently, the management of whiteflies continues to be extremely important, even if insect population is below economically damaging levels.

Other Whitefly-Transmitted Viruses Reported on Common Beans in Brazil
Although golden mosaic has been considered the main constraint on bean production in Latin America, there are other viruses transmitted by whiteflies that may cause yield losses (Fig. 16).
Cowpea mild mottle virus (CPMMV). CPMMV (family Betaflexiviridae, genus Carlavirus) is a filamentous (Fig. 17) virus, ~650 nm long, with a positive single-stranded RNA genome (King et al. 2012). In nature, CPMMV is transmitted in a non-persistent manner by the whitefly B. tabaci (Iwaki et al. 1982; Muniyappa and Reddy 1983).
 |
 |
Fig. 16. Distribution of whitefly-transmitted viruses of common beans in Brazil. BGMV = Bean golden mosaic virus; MaYSV = Macroptilium yellow spot virus; CPMMV = Cowpea mild mottle virus; SimMV = Side micrantha mosaic virus. |
Fig. 17. Electron micrograph of negatively stained Cowpea mild mottle virus particles in a leaf extract. |
 |
 |
Fig. 18. Angular mosaic symptom of Cowpea mild mottle virus on cv. Jalo Precoce 54 days after inoculation. |
Fig. 19. Initial symptoms of Cowpea mild mottle virus on mechanically inoculated cv. Jalo Precoce. |
The first occurrence of CPMMV was in Eastern Ghana in cowpeas (Vigna unguiculata) in the 1970s (Brunt and Kenten 1973). Nearly a decade later, CPMMV was reported in soybeans with symptoms of leaf crinkling and mosaic in Thailand (Iwaki et al. 1982) and in the Ivory Coast (Thouvenel et al. 1982).
CPMMV was first recorded in Brazil infecting common beans cv. Jalo in São Paulo and Paraná in 1979. Infected plants displayed typical angular yellow mosaic (Fig. 18) in the leaves of adult plants, and yield losses were considered unimportant (Gaspar et al. 1985). In 2013, CPMMV re-emerged in common beans and has frequently been detected in common beans in central and southern Brazil, becoming highly noticeable in the BGMV-resistant Embrapa GM common bean lines (Fig. 19) because of the absence of golden mosaic symptom. Infected plants of some lines drew attention to the presence of crinkled leaves often associated with vein enations visible as necrotic veins on the abaxial surface of the leaf (Fig. 20). In young inoculated plants, most common symptoms are a mild chlorosis along or between the veins, which may or may not be accompanied by a green banding of the veins. As a group, these latter symptoms are referred to as mottling, hence the virus name “mild mottle” (Fig. 21 and 22).
 |
 |
Fig. 20. Advanced mottling symptom of Cowpea mild mottle virus on cv. Jalo Precoce 34 days after inoculation.
|
Fig. 21. Bean field of the transgenic Bean golden mosaic virus-immune line BRS FC401RMD infected by Cowpea mild mottle virus under severe whitefly infestation. |
 |
Fig. 22. Detail of the abaxial side of an individual leaflet from the field of Fig. 9 exhibiting severe vein crinkling symptomatology. |
Electron microscope (EM) studies of infected leaves presented feather-like aggregates of CPMMV virions (Fig. 23) unique for whitefly-borne carlaviruses; ELISA tests were positive with CPMMV antisera kindly supplied by Almeida et al. (2005). Next generation sequence (NGS) corroborated EM and ELISA results. Double-stranded RNA was extracted from symptomatic bean plants and sequenced in an Illumina MiSeq system. A contig of 8194 nucleotides was assembled and had 93 to 98% sequence identity with six CPMMV isolates from soybean. Based on the CPMMV sequence retrieved from the NGS analysis we have designed primers suitable for PCR-based CPMMV diagnosis. Primers were used to detect CPMMV in common beans and associated weeds from different regions of the Brazilian northeastern state of Pernambuco. We confirmed the high incidence and wide distribution of CPMMV in beans and identified Macroptillium spp., Blaivillea rhomboidea, Cleome affinis, Rhynchosia minima, Senna spp., and Sida spp. as alternative hosts in northeastern Brazil. This widespread distribution of CPMMV in common beans in Brazil is troublesome. Its occurrence in combination with BGMV makes the study of yield losses difficult to undertake under field conditions. An initial evaluation of 163 accessions of Carioca-type germplasm, including commercial varieties and breeding lines, was carried out using mechanical inoculation of seedlings at the primary leaves stage under greenhouse conditions. None of the evaluated germplasm showed either immunity or hypersensitive reaction to the virus, as happens in selected soybean germplasm.
 |
Fig. 23. Transmission electron micrograph of CPMMV-infected bean leaf cell. Left: feather-like aggregate of CPMMV virions close to a nucleus. Right: similar inclusion, immunogold labeled after being exposed to anti-CPMMV antibody. Circles indicate the gold particles specifically attached to the filamentous particles forming the feather-like inclusion. |
During the soybean-growing season in 2000-2001, plants exhibiting symptoms such as stem necrosis and dwarfing were first noticed in the State of Goiás, in Central-West Brazil. Since 2001, the disease has spread to the main soybean growing areas in the states of Mato Grosso, Bahia, Maranhão, Minas Gerais, and Paraná where it has affected this crop (Almeida et al. 2005). The causal agent was characterized and shown to be CPMMV (Zanardo et al. 2014a). Host response, genome variability, and phylogenetic studies of different isolates suggest the occurrence of two distinct CPMMV strains circulating in Brazilian soybean fields associated with different biological properties and symptomatology (Zanardo et al. 2014b).
One whitefly can transmit CPMMV to bean or soybean plants with an average efficiency of 11.7%. This efficiency increased to 88.0% with 20 insects per plant. For efficient transmission, a 15-min acquisition period and a 5-min inoculation time are necessary.
Costa et al (1983) did not observe yield reduction for the inoculated plants of the Carioca seed type; however, the cv. Jalo showed yield reduction of up to 31%. Yield losses from 85 to 100% were recorded in fields cultivated with highly susceptible (those which develop necrosis) soybean cultivars (Almeida et al. 2003).
Seed transmission appears to be dependent on the viral isolate. For a Ghanaian isolate of CPMMV, seed transmission occurred in soybean and cowpea and with lower frequency in common bean (Brunt and Kenten 1973). In Venezuela, it was demonstrated that CPMMV can be transmitted by yardlong bean (Vigna unguiculata subsp. sesquipedalis) seeds (Brito et al. 2012), but Almeida et al. (2005) reported that a Brazilian CPMMV isolate was not seed-transmitted in soybean.
Almeida et al. (2003) screened soybean germplasm and identified several cultivars with tolerance to CPMMV. At least two distinct major genes determining tolerance to CPMMV in soybean were identified as having a predominance of additive genetic effects and heritability levels high enough for efficient selection (Arias et al. 2015).
Sida micrantha mosaic virus (SimMV). SimMV (family Geminiviridae, genus Begomovirus), like all viruses from the Geminiviridae family, has a single-stranded DNA (ssDNA) genome and a twinned-shaped particle constituted by the partial fusion of two icosahedral particles. SimMV was originally described in Sida micrantha plants with mosaic symptoms that were collected in Brazil in the 1960s (Jovel et al. 2004). It is found frequently infecting Sida spp. plants in diverse Brazilian regions, especially in degraded areas and those changed by human use in both rural and urban zones. SiMMV is also commonly found in central and southeastern Brazil causing mosaic and leaf distortion in soybeans (Fernandes et al. 2009). It has also been isolated occasionally from okra (Abelmascus esculentum) (Aranha et al. 2011), chili (Capsicum chilense), and passion fruit (Passiflora edulis). An isolate of SiMMV was recovered from a snap bean plant also affected by golden mosaic collected in the state of Goiás. The isolate was infectious to diverse bean and soybean cultivars (Fernandes-Acioli et al. 2011). However, SiMMV has not yet been reported from beans in other areas.
Macroptilium yellow spot virus (MaYSV). MaYSV (family Geminiviridae, genus Begomovirus) is a legume-infecting begomovirus quite divergent from BGMV. Sequence nucleotide identities among DNA-A of diverse MaYSV and BGMV isolates vary from 79.4 to 81.6%. MaYSV frequently is found naturally infecting leguminous weeds such as Macroptilium spp., Calopogonium mucunoides, Canavalia spp., and Desmodium glabrum in the northeastern Brazilian states of Alagoas, Paraiba, Pernambuco, and Sergipe (Fontenele et al. 2016; Ramos Sobrinho et al. 2014; Silva et al. 2012). MaYSV likewise has been isolated and cloned from common beans and lima beans (P. lunatus) in samples collected from the same regions (Ramos Sobrinho et al. 2014). In fact, in recent years, MaYSV seems to be displacing BGMV in both lima beans (Ramos Sobrinho et al. 2014) and common beans in these areas. In a begomovirus survey carried out in common beans in 2013 in the state of Pernambuco, 38 plants were positive for MaYSV, whereas only one plant was infected by BGMV; moreover, it was a mixed infection with MaYSV. In contrast, MaYSV has not been recorded in bean crops in the most important bean-producing areas of Brazil, situated in the Central-West, South, and Southeast regions; in these areas, BGMV is the prevalent virus in beans (Ramos-Sobrinho et al. 2014).
We succeeded in obtaining infectious MaYSV clones isolated both from common bean and Macroptilium plants. Inoculation of these clones by particle bombardment into ‘Olathe Pinto’ beans caused golden mosaic and leaf distortion. MaYSV clones were also infectious to soybeans.
The region of the rep gene used to generate the BGMV-resistant GM bean plants has low nucleotide sequence identity with the corresponding gene of MaYSV, being always below 70%. When inoculated with MaYSV infectious clones by particle bombardment, GM bean line 5.1 developed golden mosaic and leaf distortion symptoms similar to the usual symptoms displayed by non-transgenic ‘Olathe Pinto’ beans. This outcome was expected since it is well-known in the literature that one of the disadvantages of RNAi-mediated resistance is that it is not effective against viruses that differ by more than 10% from the nucleotide sequence of the transgene (Fuentes et al. 2016; Prins et al. 2008).
Whitefly-Borne Disease and Insect Management
Historically, the control of insect-transmitted viruses when the vector reaches high population levels has been difficult to achieve. In addition, the continuous migration of whitefly populations from crops such as soybeans to recently sown common beans reduces the efficiency of the attempts for chemical control. In the cases of BGMV and CPMMV, the start of the epidemics depends exclusively on the migration of viruliferous whiteflies, since there are no reports of seed transmissibility.
Chemical control. The main control method for whiteflies is still the use of synthetic insecticides. There are at least 23 registered active ingredients for this purpose in Brazil, with few showing reasonable efficiency. Their use under tropical conditions, sprayed many times within a given crop and during the whole year, likely have led to the selection of individuals in a population which are resistant to the several chemistry groups (Omer et al. 1993a,b; Cahill et al. 1996; Elbert and Nauen 2000). Thus, the rotation of insecticides with differing modes of action is critical for the prevention or at least delay of insecticide resistance development.
To avoid damage by whitefly-transmitted viruses in areas in which there is a history of high whitefly populations in the dry-season plantations or fields near soybean crops, it is important to protect the crop for 30 to 40 days after emergence. The earlier the infection by BGMV or CPMMV, the greater the yield reduction. Therefore, seed treatment is essential, followed by five to six sprays starting with 80% seedling emergence, and then every five days. Special care should be observed because some insecticides will only affect the adult population. This means that to prevent the nymphs from perpetuating the population it is also necessary to deal with this insect stage. The B. tabaci life cycle is 25 days on beans, and therefore to deal with the nymphs the control must be done about 10 days after plant emergence (egg to first instar nymph lasts 7 to 8 days). As the whitefly populations are reduced, there is a tendency to reduce adult migration from the older crops to young ones.
Cropping system practices. Most of the cultivated species within the agricultural production system in Brazil are hosts to whitefly, because it colonizes over 600 plant species (Mound and Halsey 1978; Oliveira et al. 2001; Secker et al. 1998) in the tropics, subtropics, or other mild climates without freezing temperatures. Whiteflies are referred to as polyphagous. Therefore, a vegetation-free period is an important strategy in reducing whitefly population. The only host (other than common bean) for both whitefly and the viruses BGMV and CPMMV grown on a large scale is the soybean. For this reason, the strategy most often implemented is to remove any plant live material surviving between the soybean harvest and the planting of common beans. It is particularly important to destroy any soybean volunteer plants, as they are hosts for both BGMV, CPMMV, and occasionally other whitefly-transmitted viruses.
Planting time. With the above paragraph in mind, along with the use of a host-free period, successive sowing of crops should be avoided. Good management practice would appear to include a sowing calendar for each growing region and/or crop to concentrate the major crops within a short window of seeding time.
The bean crop of the “second growing season” or “dry season,” usually from mid-February to mid-March (representing an average of 38.0% of the total production), is the main target both for BGMV and CPMMV epidemics and whitefly control. The bean crop following the host-free period is usually less affected by BGMV due to a smaller population of the vector. The winter crop is also less prone to infection because the whitefly population is greatly reduced with the end of the soybean crop season. Common bean crops should be planted at locations as far away from soybeans as possible, or a time interval should be maintained between the end of the soybean and the start of the common bean crops.
Whitefly host-free period. A host-free period for common beans was established through the initiative of growers with the support of government agencies after yield losses of about 69% in 2012-2013 due to whitefly and BGMV. The use of a whitefly host-free period has succeeded in reducing the number of insecticide applications for whitefly control and the number of bean crops lost to high incidence of BGMV.
Future Perspectives
BGMV transgenic resistance is recognized as a good example of applying biotechnology tools for the improvement of agricultural sustainability and food security for a non-industrial crop. This achievement brings with it new opportunities for pest management of all whitefly-transmitted viruses, including an integration of pest management combining the four strategies above in crops such as common beans, soybeans, cotton, maize, and tomato, which are good hosts for whiteflies. Biotechnological methods that would challenge the reproductive system used by whiteflies would be very welcome. The fact that there are conserved amino acids in the coat protein of all whitefly-transmitted begomoviruses may universalize receptor(s) present in the vector and can be a clue to finding new ways for insect or virus control, but such a receptor has not been identified as yet. Meanwhile, interfering with the viability of whitefly progenies by RNAi mechanisms of selected sequences or genes may also be a promising route, besides using bacteria or viruses which may interfere with the viability of whitefly reproduction.
References
Abel, P. P., Nelson, R. S., De, B., Hoffmann, N., Rogers, S. G., Fraley, R. T., and Beachy, R. N. 1986. Delay of disease development in transgenic plants that express the tobacco mosaic virus coat protein gene. Science 232:738-743.
Almeida, A. M. R., Piuga, F. F., Marin, S. R. R., Kitajima, E. W., Gaspar, J. O., Oliveira, T. G., and Moraes, T. G. 2005. Detection and partial characterization of a carlavirus causing stem necrosis of soybean in Brazil. Fitopatol. Bras. 30:191-194.
Almeida, A. M. R., Piuga, F. F., Kitajima, E. W., Gaspar, J. O., Valentin, N., Benato, L. C., Marin, S. R. R., Binneck, E., Oliveira, T. G., Belintani, P., Guerzoni, R. A., Nunes, J., Hoffmann, L., Nora, P. S., Nepomuceno, A. L., Meyer, M. C., and Almeida, L. A. 2003. Necrose da haste da soja. Embrapa Soja: Londrina, PR, Brazil.
Aragão, F. J. L., and Faria, J. C. 2009. First transgenic geminivirus-resistant plant in the field. Nat. Biotechnol. 27:1086-1088.
Aragão, F. J. L., and Faria, J. C. 2010. Proposta de liberação comercial de feijoeiro geneticamente modificado resistente ao mosaico dourado. Evento Embrapa 5.1 (EMB-PV051-1), CTNBio, http://ctnbio.mcti.gov.br/liberacao-comercial#/liberacao-comercial/consultar-processo.
Aragão, F. J. L., and Rech, E. L. 2001. Transgenic common bean (Phaseolus vulgaris). Pages 269-283 in: Biotechnology in Agriculture and Forestry -Transgenic Crops II. Y. P. S. Bajaj, ed. Springer-Verlag, Berlin.
Aragão, F. J. L., Ribeiro, S. G., Barros, L. M. G., Brasileiro, A. C. M., Maxwell, D. P., Rech, E. L., and Faria, J. C. 1998. Transgenic beans (Phaseolus vulgaris L.) engineered to express viral antisense RNAs showed delayed and attenuated symptoms of bean golden mosaic geminivirus. Mol. Breed. 4:491-499.
Aragão, F. J. L., Faria, J. C., Peloso, M. J., Melo, L. C., and Brondani, R. P. V. 2008. Common bean. Pages 1-22 in: Compendium of Transgenic Crop Plants. C. Kole and T. C. Hall., eds. Wiley-Blackwell, Boston, MA.
Aragão, F. J. L., Nogueira, E. O. P. E. L., Tinoco, M. L. P., and Faria, J. C. 2013. Molecular characterization of the first commercial transgenic common bean immune to the Bean golden mosaic virus. J. Biotechnol. 166:42-50.
Aranha, S. D. A., Albuquerque, L. C., Boiteux, L. S., and Inoue-Nagata, A. K. 2011. Detection and complete genome characterization of a begomovirus infecting okra (Abelmoschus esculentus) in Brazil. Trop. Plant Pathol. 36:14-20.
Arias, C. A. A., Almeida, A. M., Mituti, T., and Kitajima, E. W. 2015. Inheritance of tolerance to Cowpea mild mottle virus in soybean. Crop Breed. Appl. Biotechnol. 15:132-138.
Barbosa, L. F., Yuki, V. A., Marubayashi, J. M., De Marchi, B. R., Perini, F. L., Pavan, M. A., Barros, D. R., Ghanim, M., Moriones, E., Navas-Castillo, J., and Krause-Sakate, R. 2015. First report of Bemisia tabaci Mediterranean (Q biotype) species in Brazil. Pest Manage. Sci. 71:501-504.
Beachy, R. N. 1999. Coat–protein–mediated resistance to tobacco mosaic virus: discovery mechanisms and exploitation. Phil. Trans. R. Soc. Lond. B 354:659-664.
Bianchini, A. 1999. Resistance to bean golden mosaic virus in bean genotypes. Plant Dis. 83:615-620.
Bonfim, K., Faria, J. C., Nogueira, E. O. P. L., Mendes, E. A., and Aragão, F. J. L. 2007. RNAi-mediated resistance to bean golden mosaic virus in genetically engineered common bean (Phaseolus vulgaris). Mol. Plant-Microbe Interact. 20:717-726.
Brito, M., Rodriguez, T. F., Garrido, M. J., Mejias, A., Romano, M., and Marys, E. 2012. First report of Cowpea mild mottle Carla víruson yard long bean (Vigna unguiculata subsp. sesquipedalis) in Venezuela. Viruses 4:3804-3811.
Broughton, W. J., Hernández, G., Blair, M., Beeb, S., Gepts, P., and Vanderleyden, J. 2003. Beans (Phaseolus spp.) – model food legumes. Plant Soil 252:55-128.
Brown, J. K., Zerbini, F. M., Navas-Castillo, J., Moriones, E., Ramos Sobrinho, R., Silva, J. C. F., Briddon, R. W., Hernandez-Zepeda, C., Idris, A. M., Malathi, V. G., Martin, D. P., Rivera-Bustamante, R., Ueda, S., and Varsani, A. 2015. Revision of Begomovirus taxonomy based on pairwise sequence comparisons. Arch. Virol. 160:1593-1619.
Brunt, A. A., and Kenten, R. H. 1973. Cowpea mild mottle, a newly recognized virus infecting cowpeas (Vigna unguiculata) in Ghana. Ann. Appl. Biol. 74:67-74.
Cahill, M., Gorman, K., Day, S., Denholm, I., Elbert, A., and Nauen, R. 1996. Baseline determination and detection of resistance to imidacloprid in Bemisia tabaci (Homoptera: Aleyrodidae). Bull. Entomol. Res. 86:343-349.
Carvalho, J. L. V., Santos, J. O., Conte, C., Pacheco, S., Nogueira, E. O. P. E. L. Souza, T. L. P. O., Faria, J. C., and Aragão, F. J. L. 2015. Comparative analysis of nutritional compositions of transgenic RNAi-mediated virus-resistant bean (event EMB-PV051-1) with its non-transgenic counterpart. Transgenic Res. 24:813-819.
Costa, A. S., 1937. Nota sobre o mosaic do algodoeiro. Rev. Agric. Piriacicaba Bras. 12:453-470.
Costa, A. S. 1965. Three whitefly-transmitted virus diseases of beans in São Paulo, Brazil. FAO Plant Prot. Bull. 13:121-130.
Costa, A. S. 1975. Increase in the population density of Bemisia tabaci, a threat of widespread virus infection of legume crops in Brazil. Pages 27-49 in: Tropical Disease of Legumes. J. Bird and K. Maramorosh, eds. Academic Press, New York.
Costa, A. S. 1976. Whitefly-transmitted plant diseases. Annu. Rev. Phytopathol. 16:429-447.
Costa, A. S., Gaspar, J. O., and Vega, J. 1983. Mosaico angular do feijoeiro Jalo causado por um “carlavirus” transmitido pela mosca branca Bemisia tabaci. Fitopatol. Bras. 8:325-337.
De Barro, P. J., Liu, S., Boykin, L. M., and Dinsdale, A. B. 2011. Bemisia tabaci: A statement of species status. Annu. Rev. Entomol. 56:1-19.
Dinsdale, A., Cook, L., Riginos, C., Buckley, Y. M., and De Barro, P. 2010. Refined global analysis of Bemisia tabaci (Hemiptera: Sternorrhyncha: Aleyrodidae: Aleyrodidae) mitochondrial cytochromeoxidase to identify species level genetic boundaries. Ann. Entomol. Soc. Am. 103:196-208.
Elbert, A., and Nauen, R. 2000. Resistance of Bemisia tabaci (Homoptera: Aleyrodidae) to insecticides in southern Spain with special reference to neonicotinoids. Pest Manage. Sci. 56:60-64.
Faria, J. C., and Maxwell, D. P. 1999. Variability in geminivirus isolates associated with Phaseolus spp. in Brazil. Phytopathology 89:262-268.
Faria, J. C., Albino, M. M. C., Dias, B. B. A., Cançado, L. J., Cunha, N. B., Silva, L. M., Viana, G. R., and Aragão, F. J. L. 2006. Partial resistance to bean golden mosaic virus in a transgenic common bean (Phaseolus vulgaris) line expressing a mutant rep gene. Plant Sci. 171:565-571.
Faria, J. C., Valdisser, P. A. M. R., Nogueira, E. O. P. L., and Aragão, F. J. L. 2014. RNAi-based Bean golden mosaic virus-resistant common bean (Embrapa 5.1) shows simple inheritance for both transgene and disease resistance. Plant Breed. 133:649-653.
Feijão: dados conjunturais do feijão (área, produção e rendimento) (Phaseolus vulgaris L.) no Brasil - 1985 a 2014. http://www.cnpaf.embrapa.br/socioeconomia/index.htm
Fernandes, F. R., Cruz, A. R., Faria, J. C., Zerbini, F. M., and Aragão, F. J. 2009. Three distinct begomoviruses associated with soybean in central Brazil. Arch. Virol. 154:1567-1570.
Fernandes-Acioli, N. A. N., Pereira-Carvalho, R. C., Fontenele, R. S., Lacorte, C., Ribeiro, S. G., Fonseca, M. E. N., and Boiteux, L. S. 2011. First report of Sida micrantha mosaic virus in Phaseolus vulgaris in Brazil. Plant Dis. 95:1196.
Fontenele, R. F., Poppiel, R., Matos, V. O. L., Costa, A. F., Faria, J. C., and Ribeiro, S. G. 2016. First report of Macroptilium yellow spot virus in Desmodium glabrum in Brazil. Plant Dis. 100:657.
Fuentes, A., Carlos, N., Ruiz, Y., Callard, D., Sanchez, Y., Ochagavia, M. E., Seguin, J., Malpica-Lopez, N., Hohn, T., Lecca, M. R., Perez, R., Doreste, V., Rehrauer, H., Farinelli, L., Pujol, M., and Pooggin, M. M. 2016. Field trial and molecular characterization of RNAi-transgenic tomato plants that exhibit resistance to tomato yellow leaf curl geminivirus. Mol. Plant-Microbe Interact. 29:197-209.
Garrido-Ramirez, E. R., Sudashana, M. R., and Gilbertson, R. L. 2000. Bean golden yellow mosaic vírus from Chiapas, México: Characterization, pseudorecombination with other bean infecting geminiviruses and germplasm screening. Phytopathology 90:1224-1232.
Gaspar, J. O., Beriam, L. O. S., Alves, M. N., Oliveira, A. R., and Costa, A. S. 1985. Serological identity of bean angular mosaic and Cowpea mild mottle viruses. Fitopatol. Bras. 10:195-199.
Gilbertson, R. L., Faria, J. C., Ahlquist, P., and Maxwell, D. P. 1993. Genetic diversity in geminiviruses causing bean golden mosaic disease: The nucleotide-sequence of the infectious cloned DNA components of a Brazilian isolate of bean golden mosaic geminivirus. Phytopathology 83:709-715.
Gill, R., and Brown, J. K. 2010. Systematics of Bemisia and Bemisia relatives: Can molecular techniques solve the Bemisia tabaci complex conundrum—a Taxonomist’s viewpoint. Pages 5-29 in: Bemisia: bionomics and management of a global pest. P. A. Stansly, and S. E. Naranjo, eds. Springer, Dordrecht, The Netherlands.
Gonsalves, D., Gonsalves, C., Ferreira, S., Pitz, K., Fitch, M., Manshardt, R., and Slighton, J. 2004. Transgenic virus-resistant papaya: From hope to reality for controlling papaya ringspot vírus in Hawaii. (http://www.apsnet.org/publications/ apsnetfeatures/Pages/papayaringspot.aspx).
Iwaki, M., Thongmeearkon, P., Prommin, M., Honda, Y., and Hibi, J. 1982. Whitefly transmission and some properties of Cowpea mild mottle virus on soybean in Thailand. Plant Dis. 66:265-268.
Jovel, J., Reski, G., Rothenstein, D., Ringel, M., Frischmuth, T., and Jeske, H. 2004. Sida micrantha mosaic is associated with a complex infection of begomoviruses different from Abutilon mosaic virus. Arch. Virol. 149:829-841.
King, A. M. Q., Lefkowitz, E., Adams, M. J., and Carstensand, E. B. 2012. Virus taxonomy: Ninth report of the International Committee on Taxonomy of Viruses, 9th ed. Elsevier, San Diego, CA.
Lima, A. T. M., Sobrinho, R. R., González-Aguilera, J., Rocha, C. S., Silva, S. J. C., Xavier, C. A. D., Silva, F. N., Duffy, S., and Zerbini, F. M. 2013. Synonymous site variation due to recombination explains higher genetic variability in begomovirus populations infecting noncultivated hosts. J. Gen. Virol. 94:418-431.
Lourenção, A. L., and Nagai, H. 1994. Surtos populacionais de Bemisia tabaci no Estado de São Paulo. Bragantia 53:53-59.
Morales, F. J., and Anderson, P. K. 2001. The emergence and dissemination of whitefly-transmitted geminiviruses in Latin America. Arch. Virol. 146:415-441.
Mound, L. A., and Halsey, S. H. 1978. Whitefly of the world: A systematic catalogue of the Aleyrodidae (Homoptera) with host plant and natural enemy data. J. Wiley, Chichester, UK.
Muniyappa, V., and Reddy, D. V. R. 1983. Transmission of Cowpea mild mottle virus by Bemisia tabaci in a non persistent manner. Plant Dis. 67:391-393.
Oliveira, M. R. V., Henneberry, T. J., and Anderson, P. 2001. History, current status and collaborative research projects for Bemisia tabaci. Crop Prot. 20:709-723.
Omer, A. D., Tabashnik, B. E., Johnson, M. W., and Leigh, T. F. 1993a. Realized heritability of resistance to dicrotophos in greenhouse whitefly. Entomol. Exp. Appl. 68:211-217.
Omer, A. D., Johnson, M. W., Tabashnik, B. E., Costa, H. S., and Ullman, D. E. 1993b. Sweetpotato whitefly resistance to insecticides in Hawaii: Intra-island variation is related to insecticide use. Entomol. Exp. Appl. 67:173-182.
Paula, N. T., Faria, J. C., and Aragão, F. J. L. 2015. Reduction of viral load in whitefly (Bemisia tabaci Gen.) feeding on RNAi-mediated bean golden mosaic virus resistant transgenic bean plants. Virus Res. 210:245-247.
Pessoni, L. A., Zimmermann, M. J. O., and Faria, J. C. 1997. Genetic control of characters associated with bean gold mosaic geminivirus resistance in Phaseolus vulgaris L. Braz. J. Genet. 20:51-58.
Prins, M., Laimer, M., Noris, E., Schubert, J., Wassenegger, M., and Tepfer, M. 2008. Strategies for antiviral resistance in transgenic plants. Mol. Plant Pathol. 9:73-83.
Quintela, E. D., Abreu, A. G., Lima, J. F. S., Mascarin, G. M., Santos, J. B., and Brown, J. K. 2016. Reproduction of the whitefly Bemisia tabaci (Hemiptera: Aleyrodidae) B biotype in maize fields (Zea mays L.) in Brazil. Pest Manage. Sci. doi:10.1002/ps.4259.
Ramos Sobrinho, R., Xavier, C. A., Pereira, H. M., Lima, G. S., Assunção, I. P., Mizubuti, E. S., Duffy, S., and Zerbini, F. M. 2014. Contrasting genetic structure between two begomoviruses infecting the same leguminous hosts. J. Gen. Virol. 95:2540-2552.
Rosen, R., Kanakala, S., Kliot, A., Pakkianathan, B. C., Farich, B. A., Santana-Magal, N., Elimelech, M., Kontsedalov, S., Lebedev, G., Cilia, M., and Ghanim, M. 2015. Persistent, circulative transmission of begomoviruses by whitefly vectors. Curr. Opin. Virol. 15:1-8.
Secker, A. E., Bedford, I. A., Markham, P. G., and Willlam, M. E. C. 1998. Squash, a reliable field indicator for the presence of B biotype of tabaco whitefly, Bemísía tabací. Pages 837-842 in: Proc. of the Brighton Crop Prot. Conf.: Pests and Dis., Brighton, UK, 16-19 Nov. 1998.
Silva, S. J. C., Castillo-Urquiza, G. P., Hora, B. T., Assunção, I. P., Lima, G. S. A., Pio-Ribeiro, G., Mizubuti, E. S. G., and Zerbini, F. M. 2012. Species diversity, phylogeny and genetic variability of begomovirus populations infecting leguminous weeds in northeastern Brazil. Plant Pathol. 61:457-467.
Stansly, P. A., and Naranjo, S. E., eds. 2010. Bemisia: Bionomics and Management of a Global Pest. Springer, Dordrecht, The Netherlands.
Thouvenel, J. C., Monsarrat, A., and Fauquet, C. M. 1982. Isolation of Cowpea mild mottle virus from diseased soybean in the Ivory Coast. Plant Dis 66:336-337.
Tulmann Neto, A., Ando, A., and Costa, A. S. 1977. Attempts to induce mutants resistant or tolerant to golden mosaic virus in dry beans (Phaseolus vulgaris L.). Pages 281-288 in: IAEA, Induced Mutation Against Plant Diseases, IAEA Proceedings Series.
Zanardo, L. G., Silva, F. N., Bicalho, A. A. C., Urquiza, G. P. C., Lima, A. T. M., Almeida, A. M. R., Zerbini, F. M., and Carvalho, C. M. 2014a. Molecular and biological characterization of Cowpea mild mottle virus isolates infecting soybean in Brazil and evidence of recombination. Plant Pathol. 63:456-465.
Zanardo, L. G., Silva, F. N., Lima, A. T., Milanesi, D. F., Castilho-Urquiza, G. P., Almeida, A. M., Zerbini, F. M., and Carvalho, C. M. 2014b. Molecular variability of Cowpea mild mottle virus infecting soybean in Brazil. Arch. Virol. 159:727-737.