Introduction
Human efforts to produce ever-greater amounts of food reached a historic pinnacle in 1981 (11), and a landmark 1991 United Nations study estimated that soil in 552 million hectares of land, equal to 38% of today’s global cultivated area, had been degraded to some degree by agricultural mismanagement since WWII (11). Although the use of modern agricultural technologies to increase yield has become an effective substitute for land expansion, global agroecosystems are becoming more vulnerable to supply failures than when expansion to new land areas was an option. These issues have increased public awareness of soil ecology and the importance of maintaining soil health in agroecosystems.
Definition of Soil Health
Soil health is the capacity of a soil to function within ecosystem boundaries to sustain biological productivity, maintain environmental quality, and promote plant and animal health (4). A healthy soil should be able to support life processes such as plant anchorage and nutrient supply, retain optimal water and soil properties, support soil food webs, recycle nutrients, maintain microbial diversity, remediate pollutants, and sequester heavy metals. Plant pathologists assert that disease suppression also should be a function of soil health.
In this article, we adopt all these definitions of soil health and synthesize a perspective relating to nematode management. While conventional nematode management focuses on suppressing plant-parasitic nematodes, we propose to also manage the more abundant and beneficial free-living nematodes in the soil ecosystem. This is feasible because of comprehensive nematode faunal analysis studies that have been conducted over the last few decades (1,6,7,8,13).
Our approach is to develop soil ecosystem management strategies to protect against damage caused by plant-parasitic nematodes ultimately leading to improvements in plant health. The characteristics of soil health emphasized include: high biological diversity, high community stability that can provide resilience to disturbance (short-term) or stress (long-term), ability to maintain the integrity of nutrient cycling and energy flow, suppression of multiple pests and pathogens, and improved plant health.
Background on Nematodes as Soil Health Bioindicators
Nematodes can be used as bioindicators of soil health because they are ubiquitous and have diverse feeding behaviors and life strategies, ranging from colonizers to persisters. Some nematodes can survive harsh, polluted, or disturbed environments better than others, and some have short life cycles and respond to environmental changes rapidly. In general, nematodes are easy to sample and extract from soil, their morphology reflects feeding behavior allowing easy functional classification, and nematode taxa are well classified (2,17). Because of these characteristics, nematode faunal analysis provides insight into soil food web conditions.
Although there are some ambiguities in feeding behavior for some nematode species, soil nematodes were classified into eight feeding groups (27). Five of the most common feeding groups with very distinct buccal (mouth) cavities are shown in Figure 1. A conceptual advance in nematode community analysis was the classification of nematode families on a colonizer-persister (c-p) continuum of 1 to 5 (1). Nematodes with a c-p value equal to one are short lived, have high fecundity, feed in enriched media, and form dauer larvae as microbial blooms subside; whereas those with c-p values of five are long lived, have larger bodies, low fecundity, the greatest sensitivity to disturbance, and are predominantly omnivores or predators (1,2). Taxa in the same c-p classes respond to disturbance similarly. The maturity index (MI), a calculation of the weighted mean frequency of the c-p-scaling across the entire nematode community, provides a representation of the nematode community and presumably of the condition of the soil environment (1).
|
 A |
|
|
 E |
|
Fig. 1. Buccal cavities of five nematode feeding groups:
(A) bacterivore; (B) fungivore; (C) herbivore; (D) omnivore, and; (E) predator.
The combination of nematode feeding groups and c-p-scaling into functional guilds (2) has developed nematode faunal analysis into a more powerful tool which can be used as a bioindicator of soil health and food web condition (6). Ferris et al. (6) used functional guilds to compute an enrichment index (EI), and a structure index (SI). The EI is based on the expected responsiveness of the opportunistic guilds (bacterivores with c-p = 1 or Ba1) to food resource enrichment. Thus, EI describes whether a soil ecosystem is nutrient enriched (high EI) or depleted (low EI). The SI represents an aggregation of functional guilds with c-p values ranging from 3-5. Structure index describes whether a soil ecosystem is structured/matured (high SI) or disturbed/degraded (low SI) (Fig. 2). In addition, Ferris et al. (6) proposed a channel index (CI), which is the percentage of fungivores among the total of fungivores and opportunistic bacterivores to describe the dominant decomposition channels in a food web. Such analyses provide slightly different information than analyses based on biomass, diversity, and plant-parasitic species, and take into consideration both descriptive and quantitative information on soil ecosystems (1,7).
|
Fig. 2. A simplified food web structure on enrichment index (EI) and structure index (SI) trajectories (modified from Ferris et al., 2001). |
|
Many nematode ecologists throughout the world have studied the impacts of human intervention on nematode fauna and searched for sensitive bioindicators of soil health (17). In summary, using nematodes as bioindicators makes use of both biotic and abiotic factors (e.g., toxic compounds, nutrients), and provides insight into both the function and structure of the soil ecosystem.
Role of Nematodes in Soil Nutrient Cycling
Detritus and organic residues must decompose to release nutrients for plant uptake. Decomposition of organic matter in a soil food web can be divided into two energy channels, a faster bacterial channel and a slower fungal-based channel. Soil ecosystem types and nutrient forms (e.g., C:N ratios) determine the predominant decomposition channels (7,13). Although bacteria and fungi are the primary decomposers in the soil food web (Fig. 3), these microbes also can immobilize inorganic nutrients in the soil (13). As an extension of these decomposition channels, when the bacterivorous and fungivorous nematodes graze on these microbes, they give off CO2 and NH4+ and other nitrogenous compounds, affecting C and N mineralization directly (13). Indirectly, nematodes can disseminate microbial propagules throughout the soil (10), which advances the colonization of substrates and mineralization of nutrients. Nematode metabolites may also stimulate specific bacterial growth by releasing growth-limiting nutrients (such as N and vitamins). However, overgrazing of bacterial or fungal populations by nematodes can result in a reduction of the overall activity of these decomposers. Fortunately, in the hierarchy of the soil food web, generalist predators prey on these bacterivorous and fungivorous nematodes, improving nutrient cycling and allowing more nutrients to be released (28).
|
Fig. 3. Roles of nematodes in organic matter decomposition (modified from Ingham et al., 1985). |
|
Therefore, nematodes play important roles in soil nutrient cycling. Nematode excretion may contribute up to 19% of soluble N in soil (17). This is due to the fact that nematodes (C:N ratio of 8-12) have a lower N content than the bacteria (C:N ratio of 3-4) they consume (26). In addition, the growth efficiency of nematodes (< 25%) is smaller than those of the bacteria (> 30%) (12). Therefore, nematodes excrete a majority of both the assimilated C and N that they consumed from the bacteria. Bacteria, on the other hand, usually respire most of the assimilated C, but immobilized most of the assimilated N. Therefore the contribution made by nematodes to N mineralization is relatively high compared to bacteria in soil ecosystems.
Besides contributing to N mineralization, the abundance of many free-living nematodes, especially bacterivorous, omnivorous, and predatory nematodes, also were found to correlate with concentrations of many other soil nutrients in a fallow field (22), suggesting the possibility of nematodes mineralizing many other soil nutrients. More clear-cut relationships between nematodes and soil nutrients were observed in a field that had been fallow for 1.5 years compared to a recently cultivated field (22). The abundance of many bacterivorous genera followed predators and omnivores and correlated significantly (P < 0.10) with most soil nutrient concentrations. However, few significant correlations occurred with genera in fungivore and herbivore groups.
Goals in Developing Soil Ecosystem Management from a Nematode Management Perspective
Maintaining soil nutrient availability and plant-parasitic nematode suppression are two of the most important issues in nematode management for soil health. Plant-parasitic nematodes cause damage to plant roots, resulting in root systems which are less able to take up nutrients and water. Enhancing soil nutrient availability not only supplies nutrients for plant uptake, but also provides plants with materials needed to grow functional roots, thus increasing the plant tolerance to nematode damage. On the other hand, a great resource in most soil ecosystems for suppressing plant-parasitic nematodes is the pool of natural enemies of nematodes in the soil. These include fungal egg-parasites, nematode-trapping and endoparasitic fungi, plant-health promoting rhizobacteria, obligate bacterial parasites, mites, collembola, tardigrades, predatory nematodes, turbellarians, and protozoans (Fig. 4).
 A |
|
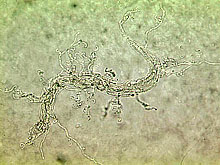 B |
|
 C |
|
Fig. 4. Some natural enemies of nematodes commonly found in soil: (A) Arthrobotrys dactyloides,
a nematode-trapping fungus, forming constricting rings to trap a root-knot nematode;
(B) Harposporium anguillulae, a nematode-endoparasitic fungus, colonizing a bacterivorous nematode;
(C) Mononchus sp., a predatory nematode.
Therefore, our goals in developing soil ecosystem nematode management systems encompass: (i) the enhancement of free-living nematodes that are significantly involved in soil nutrient cycling; (ii) the suppression of multiple nematode pests; (iii) the enhancement of natural enemies of plant-parasitic nematodes, and (iv) the improvement of plant health. Several studies of nematode communities provide useful examples of how some of these goals may be achieved.
Enhancing free-living nematodes that are significantly involved in soil nutrient cycling. Due to their efficiency in nutrient mineralization, particularly N, the abundance of bacterivorous nematodes may be a useful indicator of enhanced soil nutrient cycling (10). Ferris and Matute (7) specified that to provide a healthy soil ecosystem with adequate soil fertility, enrichment-opportunists (bacterivorous nematodes in Ba1 guild) need to be maintained at a high level. The enrichment of soil nematode communities is driven by organic input, based on the direct relationship between the cumulative amounts of N mineralized and the EI (7). In addition, Ferris and Matute (7) also found an inverse relation between the slope of CI (i.e. the rate at which decomposition changed from bacterial to fungal) and N mineralization. Thus, EI and CI could be good bioindicators of N mineralization. However, EI may also be low in non-disturbed natural ecosystems with high nutrient turnover rates, rendering this index less suitable as an indicator of soil health (7). Nonetheless, projecting nematode community structure on to EI and SI continuums may provide a useful quantitative view of soil health (Fig. 2).
Suppressing multiple nematode pests. Conventional, non-chemical management practices for plant-parasitic nematodes often target the suppression of a single nematode pest. For instance, some crop rotations or inundative biological control methods may target only a single pest. In soil ecosystem nematode management, ideally one should aim for suppressing multiple plant-parasitic nematode species. This might be difficult to achieve with a single procedure. However, the use of a cover crop that is a poor host to multiple species of plant-parasitic nematodes might be closer to this goal. For instance, sunn hemp (Crotalaria juncea), used as a cover crop, was suppressive to root-knot nematodes as well as reniform nematodes (25).
Enhance natural enemies of plant-parasitic nematodes. Some scientists believe that using soil amendments can increase the activity of free-living nematodes and enhance soil suppressiveness to plant-parasitic nematodes. Van den Boogert et al. (19) supported this hypothesis and concluded that organic matter stimulated bacteria numbers, provided a food base for free-living nematodes, which in turn became a food source for nematode-trapping fungi. Some nematode antagonistic fungi occupy the rhizosphere in preference to the general soil mass. Therefore, planting cover crops would be preferable to fallowing between crop seasons to enhance the populations and activity of these antagonists.
Parasitism of some nematode antagonistic fungi is dependent on the population densities of host nematodes, a phenomenon known as host density-dependent parasitism. However, dependency on nematodes varies among nematophagous fungi; those that produce adhesive networks appear to be less dependent on nematodes than are those that produce constricting rings and adhesive knobs (3). Jaffee (14) demonstrated that the greatest dependency on host density was observed in Hirsutella rhossiliensis (an endoparasitic fungus), intermediate for Monacrosporium ellipsosporum (a trapping fungus forming adhesive knobs) and A. dactyloides (a trapping fungus forming constricting rings), and least for A. oligospora (a trapping fungus forming adhesive three-dimensional networks) and M. cionopagum (a trapping fungus forming adhesive branches and two-dimensional adhesive networks). Therefore, it is not surprising to find higher population densities of host density-dependent nematode-antagonistic fungi in soils heavily infested with plant-parasitic nematodes.
Improve plant health. The ultimate goal of soil ecosystem management is to improve plant health. Amending soil with organic matter is a basic practice for soil ecosystem management because even though this practice does not always suppress soil pests, it still can increase crop yields, which is the main concern for a grower. In a field trial of yellow squash, amendment with yard-waste compost (very high C:N ratio, minimal nutrient release) at 269 mt/ha, either incorporated into the soil or as mulch, did not suppress M. incognita population densities at the end of the experiment. However, incorporating compost resulted in an increase of squash yield compared to the control treatment, indicating an increase in plant tolerance against plant-parasitic nematode infection, attributed to improved water-holding capacity in mulched plots (16).
Why is it Important to Maintain Soil Functional Diversity?
Stability of soil ecosystems. Microbial diversity in soil is usually assessed as species or taxa diversity. However, structural and functional diversity are more important to soil health. Functional redundancy, which refers to a reserve pool of quiescent organisms or a community with vast interspecific overlaps and trait plasticity, allows an ecosystem to maintain long-term stability of soil function. Considerable functional redundancy exists and merits protection because this may be why decomposition processes are maintained in highly disturbed soils despite the extinction of many species (5).
Succession on nematode communities. During the decomposition of organic matter with a mixture of C:N ratios (Fig. 5), populations of enrichment-opportunist bacterivorous nematodes (Ba1 guild) increased rapidly in response to low C:N materials, and to a lesser extent, to more complex materials. The general-opportunist bacterivores (Ba2 guild) increased at a slower rate. Fungivorous nematodes increased gradually as higher C:N ratio residues became more abundant, but increased most rapidly in soil amended with higher C:N and more complex materials (7). These in turn were replaced by persisters (c-p 3-5), which encompass most of the omnivores and predators (Fig. 5).
|
Fig. 5. Nematode community succession in relation to C:N ratios of soil amendments. |
|
Succession of nematodes is not limited to the trophic group level, but also occurs among taxa (genera or species) within a feeding group. In general, nematode succession follows the pattern cp-1® cp-2 ® cp-3 to 5, as demonstrated in a typical succession of nematodes after adding organic material to the soil (23). Besides the above mentioned succession from Ba1 (Rhabditidae) to Ba2 (Cephalobidae) followed by Ba3 (e.g., Euteratocephalus and Prismatolaimus) (6), Ferris et al. (9) even found that different species of bacterivores in the same guild made substantial contributions to N mineralization in different months (9), ensuring the continuum of nutrient cycling. It is possible that changes in climate among months might have great impact on this species diversity.
In addition, succession among genera in the fungivore group is also well documented. In a study with litterbags containing sunn hemp residues, we observed a succession of fungivores in the litterbags: Aphelenchus ® enchytraeid worms (which were primarily fungivores) ® other fungivorous nematodes including Filenchus, Tylenchus, and Tylencholaimus (23). Aphelenchoides, however, occurred at an intermediate level throughout the duration of residue decomposition. This is because different fungivores have their own fungal species preferences (18). Species diversity ensures that when one fungal food source is diminishing due to overgrazing, the soil food web continues to function. Without a high biological diversity, a soil ecosystem will be very vulnerable to environmental changes, disturbance or stress.
Agricultural Practices Compatible with Soil Ecosystem Management
Two major characteristics of farming systems that are compatible with soil ecosystem management are the addition of organic amendments and avoiding the application of synthetic pesticides, similar to organic farming practices. A major concern in organic systems is the maintenance of adequate soil fertility at key crop-growth periods. Availability of nutrients from an organic matter source varies substantially among different kinds of organic matter (7), mainly due to differences in C:N ratios. With low C:N ratio organic substrates, bacterial growth is C-limited, and N-immobilization by microbes will be minimal; but at high C:N ratios, bacterial growth will be N-limited, and there may be rapid immobilization of newly mineralized N. Therefore, it is critical to select an organic amendment with a low C:N ratio if rapid nutrient availability for the crop plant is needed. If high C:N ratio amendments are used, soil food webs will be selected for fungal dominated decomposition pathways, thus a slower mineralization rate but a longer lasting supply of organic materials will be available.
Example of Agricultural Practice with Multiple Benefits to Soil Health
In this article, we have illustrated the use of a specific organic amendment (sunn hemp) as a practice with multiple benefits in soil ecosystem management. Sunn hemp, Crotalaria juncea L., is a legume (Fig. 6) that is receiving increased attention due to its efficient green manure properties, including its ability to fix nitrogen, rapidly produce biomass, increase soil organic matter, and suppress many plant-parasitic nematodes (24). Sunn hemp also has been documented to increase population levels of nematode-antagonistic fungi (24) and bacterivorous nematodes involved in soil nutrient cycling, and improve soil nutrient levels (20). Therefore, it is considered a good rotation crop for organic and other sustainable agricultural systems.
 A |
|
 B |
Fig. 6. (A) Vegetative and (B) flowering stages of sunn hemp (Crotalaria juncea).
Sunn hemp residues have potential as an organic nutrient source in soil ecosystem management because they have an average C:N ratio of 18.9, but when tissues are separated, the C:N ratios are 11.3 for leaves, 11.7 for racemes, and 48.0 for stems (15). These C:N ratios allow most of the nitrogen in sunn hemp hay to mineralize readily in soil in the presence of soil microorganisms because N may be easily mineralized when the C:N ratio is less than 20:1. Additionally, since the sunn hemp hay used provides a range of C:N ratios, various resources likely become available at different times, allowing both Ba1 and Ba2 guilds to coexist and increase, ultimately providing the possibility that nutrients would be available to the plant over a longer time than if a homogenous C:N ratio material was used. The potential of sunn hemp amendments for promoting soil health is evaluated subsequently based on the criteria for soil health.
Increase in biological diversity and maintenance of nematode community stability. In a sunn hemp decomposition experiment (23), we monitored nematode community changes at two-week intervals over a 70-day period in a corn field. Litterbags containing sunn hemp residues were buried in the soil. Biological diversity was increased by sunn hemp residues as indicated by the increase in diversity and richness of nematode communities at 70 days after bag burial compared to before bag burial (Figs. 7a and b). Soil beneath the buried sunn hemp litterbags (receiving sunn hemp leachate) had reduced MI and SI initially but later returned to the same level as the no bag control treatment, thus indicating maintenance of the stability of soil communities (Figs. 7c and d).
Bacterivorous nematode population levels reached a peak 2 weeks after bag burial (Fig. 7e), coinciding with a great reduction in N content in litterbags (15), indicating the period in which nutrients were most available for plant uptake. Thus, sunn hemp amendment enriched the soil ecosystem, ensuring active nutrient cycling. The abundance of fungivorous nematode inside the sunn hemp bag peaked once at the same time as the bacterivores (at 14 DAB), but peaked again 56 days after bag burial (Fig. 7f), indicating residues with a mixture of C:N ratios were available inside the bag initially, consistent with the C:N ratio analysis of different tissues of sunn hemp (15). The increase of omnivores but not predatory nematodes at a significant level (Fig. 7g) partially supports the continuum of nematode succession in this soil ecosystem and demonstrates that nutrients and energy are transferred to a higher level in the food web. The succession of nematodes in different trophic groups after sunn hemp bag burial is consistent with enhanced soil nutrient cycling and energy flow. Thus, soil amended with sunn hemp not only provides N readily for plant uptake, but also enhances nematode grazers that can further mineralize other organic residues in soil with higher C:N ratios. The percentage of herbivorous nematodes was significantly lower in the soil below a sunn hemp litterbag compared to soil without a litterbag (Fig. 7h). This is likely due to the fact that sunn hemp leachate contained substances suppressive to many plant-parasitic nematodes (24,25).
|
Fig. 7. Effect of sunn hemp hay on nematode communities. Temporal distribution of: (a) nematode richness, and (b) diversity inside the sunn hemp bag; (c) maturity index and (d) structure index inside the sunn hemp bag (B), soil under the sunn hemp bag (SI), and soil without the sunn hemp bag (SO); numbers of (e) bacterivorous nematode, (f) fungivorous nematode, (g) omnivorous nematode inside the sunn hemp bag; and (h) percentage of herbivores in B, SI and SO. Values followed by different letters are different among sampling dates (graphs with single lines) or among samples within a sampling dates (graphs with multiple lines) at P < 0.05 according to Waller-Duncan k-ratio (k = 100) t-test. |
|
Enhance nematodes involved in nutrient cycling and availability. The ability of sunn hemp amendments to enhance nematodes involved in nutrient cycling was examined with three soils of different histories in greenhouse experiments (20). The total number of bacterivorous nematodes were increased by sunn hemp amendment in soil collected from a field with short history of vegetable crop rotation that had relatively low organic matter (OM) =1.9% and low numbers of bacterivores, but not in soils collected from long-term agricultural sites, with higher levels of OM (2.4% - 8.4%) and bacterivores. Data from these experiments suggested that the abundance of bacterivorous nematodes might reach a carrying capacity in the soil contained in greenhouse pots, such as those with long-term cropping histories that had an abundance of bacterivorous nematodes even without sunn hemp amendment. Therefore, sunn hemp amendment is able to enhance bacterivorous nematodes when the carrying capacity of this type of nematode has not been reached.
Suppression of multiple pests and pathogens. Sunn hemp amendments suppressed root-knot nematodes in a greenhouse experiment with a lower inoculum level (200 nematodes per pot) of root-knot nematodes but not in an experiment with a high inoculum level (800 nematodes per pot) (21). The high nematode inoculum might be too high for sunn hemp amendment to suppress sufficiently. However, plant growth differences between sunn hemp amended and unamended soils were greater in the experiment with high nematode inoculum than that with a lower inoculum level. A healthier plant might have resulted in higher number of root-knot nematodes in the sunn hemp amended soil. When root-knot numbers were adjusted to numbers per g of shoot weight, reduction in root-knot numbers by sunn hemp was observed even in the experiment with high nematode inoculum. Besides M. incognita, sunn hemp has been reported to be suppressive to M. javanica, M. arenaria, M. exigua, Rotylenchulus reniformis, Radopholus similis, Helicotylenchus multicinctus, and Hoplolaimus indicus (25).
Enhance natural enemies of nematodes. Although sunn hemp was shown to be able to enhance nematode antagonistic fungi after soil incorporation (24), results from two greenhouse experiments showed variable results in nematode suppression (21). Most of the nematode-antagonistic fungi in one experiment were nematode-trapping fungi including Arthrobotrys brochopaga, A. oligospora (Figs. 8a and b), A. superba, and Monacrosporium parvicollis which can prey on root-knot nematodes, whereas in the other experiment, nematode endoparasitic fungi, such as Catenaria sp. and Harposporium anguillulae (Figs. 8c and d), dominated the soil. Harposporium anguillulae can only attack bacterivorous nematodes but not root-knot nematodes. Although sunn hemp amendment enhanced both types of nematode-antagonistic fungi in these experiments, enhancement of nematode-trapping fungi might have resulted in root-knot nematode suppression, while enhancement of H. anguillulae did not affect the root-knot nematode population.
 A |
|
 B |
 C |
|
 D |
Fig. 8. Nematode trapping (A and B) and endoparasitic (C and D) fungi:
(A) Arthrobotrys brochopaga; (B) A. oligospora;
(C) Harposporium anguillulae; and (D) Catenaria sp.
Sunn hemp amendments also increased omnivorous and predatory nematodes (20), which are natural enemies to plant-parasitic nematodes. Although a significant contribution of predatory nematodes toward suppression of plant-parasitic nematodes might not be obvious, the increase in predatory nematodes may result in increased cycling of plant nutrients (28). This is because predatory nematodes are often feeding on bacterivorous or fungivorous nematodes that apparently served as conduits through which more nutrients could be released.
Increase soil nutrient availability. The sunn hemp, in whole plant form, contains N-P2O5-K2O in amounts of 138-47-90 kg/ha, for a ratio of approximately 3:1:2 (15). Therefore, sunn hemp could be an adequate fertilizer to meet most of the N, P, K nutrient requirements of vegetable crops. Since most of the N and other macronutrients are found in leaves and flower heads, use of sunn hemp as a mulch or green manure would be most beneficial at the early- to mid-flowering stages (15).
Improve in plant health. Increased plant growth following sunn hemp amendment is commonly reported for many crops (15). Incorporating 100 kg N/ha of sunn hemp hay into a squash field at planting achieved squash yield equivalent to those in plots fertilized with the same N rate of ammonium nitrate (15). In the greenhouse experiment described earlier (21), sunn hemp amendments increased shoot and root weight of squash in most of the soils tested except in a soil that already had an unusually high level of organic matter (8.4%).
Model of Soil Ecosystem Management
As demonstrated in the evaluation of sunn hemp amendments in promoting soil health, despite all the beneficial properties of sunn hemp, its performance is affected by many factors. Therefore the success of soil ecosystem management does not rely simply on the choice of agricultural practices. A model (Fig. 9) can provide a reference for managing nematodes in the context of soil ecosystem health. The model for soil ecosystem management differs from organic farming systems in that in addition to selecting agricultural practices that use organic fertilizers and avoid chemical pesticides, one also should consider monitoring communities in the soil ecosystem to decide if the practices used will promote soil health. The timing of the practices should be optimized to integrate with farmers’ cropping systems. The history of the farming site will also affect the outcome of soil ecosystem management. For example, a soil that already had high levels of organic matter and bacterivorous nematodes did not respond to addition of sunn hemp amendments (20). Some considerations in interpreting and using this model are as follows:
|
Fig. 9. Soil ecosystem model for nematode management. |
|
Practices that enhance soil health. Although an important practice that enhances soil health is the use of organic matter, the decomposition rate and products of organic materials in the soil depend on their nature and C:N ratios and the time-course of decomposition. Nitrogen may become immobilized in microbial tissue when organic material has a C:N ratio greater than 20:1 but mineralized in the form of NH4+ or NO3- when the C:N ratio is less than 20:1.
Ecosystem monitoring. Monitoring the soil ecosystem prior to the cropping season will help to determine if the practices selected are compatible with the achievement of goals of soil ecosystem management. For example, if natural enemies of major pests in a particular site are not present, biocontrol agents might be added to the soil. Since the history of agricultural practices can affect soil ecosystem, knowing the history of an agricultural site can also be helpful in making decisions. For instance, rates of cover crop decomposition are more consistent in organically managed soils than in conventionally farmed soils. In addition, the C:N ratio of the cover crop (higher for small grains, lower for legumes) will affect the rate of its decomposition. Besides monitoring biotic factors, monitoring abiotic factors in soil also will be important because nematode community structure is influenced by a combination of previous land use, and soil factors such as soil type and texture.
Timing. The timing of application of organic matter prior to crop planting differs among the specific fertilizers used. For example, in the case of using sunn hemp residue as an organic fertilizer, peak abundance of bacterivorous nematodes occurred 2 weeks after sunn hemp burial, indicating that the peak N mineralization rate also would be expected around this time period. Therefore, growers could time planting and fertilizer application so that the crop will be ready to take up the nutrients within 2 weeks after the sunn hemp residues are applied. On the other hand, when using a manure with the relatively high C:N ratio of 34.8 as a source of organic matter, it was recommended that the manure be incorporated at the end of the previous crop to increase the abundance, biomass, and activity of bacterivorous nematodes in the spring (8).
Conclusion
The concept of soil ecosystem management is still at a developmental stage. While the model provides us a general guideline toward maintaining a healthy soil ecosystem, much information must still be added to advance our understanding of soil health for ecosystem management. Interdisciplinary collaboration among scientists such as plant-pathologists, nematologists, agronomists, soil scientists, horticulturists, entomologists, weed scientists, soil ecologists, and microbiologists are necessary to broaden the application of ecosystem management.
Additional Resources
Management of Nematodes and Soil Fertility with Sunn Hemp Cover Crop, IFAS Extension
Cover Crops, IFAS
Nematophagous Fungi, IFAS
Soil Nematodes, IFAS
Literature Cited
1. Bongers, T. 1990. The maturity index: an ecological measure of environmental disturbance based on nematode species compostion. Oecologia 83:14-19.
2. Bongers, T., and Bongers, M. 1998. Functional diversity of nematodes. Appl. Soil Ecol. 10:239-251.
3. Cooke, R. C. 1963. Ecological characteristics of nematode-trapping fungi Hyphomycetes. Ann. Rev. Appl. Biol. 52:431-437.
4. Doran, J. W., Sarrantonio, M., and Liebig, M. A., eds. 1996. Soil health and sustainability, Adv. Agron. 56:1-54.
5. Ettema, C. H. 1998. Soil nematode diversity, species coexistence and ecosystem function. J. Nematol. 30:159-169.
6. Ferris, H., Bongers, T., and deGoede, R. G. M. 2001. A framework for soil food web diagnostics: Extension of the nematode faunal analysis concept. Appl. Soil Ecol. 18:13-29.
7. Ferris, H., and Matute, M. M. 2003. Structural and functional succession in the nematode fauna of a soil food web. Appl. Soil Ecol. 23:93-110.
8. Ferris, H., Venette, R. C., and Lau, S. S. 1996. Dynamics of nematode communities in tomatoes grown in conventional and organic farming systems, and their impact on soil fertility. Appl. Soil Ecol. 3:161-175.
9. Ferris, H., Venette, R. C., and Lau, S. S. 1997. Population energetics of bacterial-feeding nematodes: Carbon and nitrogen budgets. Soil Biol. Biochem. 29:1183-1194.
10. Freckman, D. W. 1988. Bacterivorous nematodes and organic-matter decomposition. Agr. Ecosyst. Environ. 24:195-217.
11. Gardner, G. 1998. Preserving global cropland. Pages 42-59 in: State of the World, 1997. L. Starke, ed. W. W. Norton and Company, New York, NY.
12. Hunt, H. W., Coleman, D. C., Ingham, E. R., Elliott, E. T., Moore, J. C., Rose, S. L., Reid, C. P. P., and Morley, C. R. 1987. The detrital foodweb in a shortgrass prairie. Biol. Fertil. Soils 3:57-68.
13. Ingham, R. E., Trofymow, J. A., Ingham, E. R., and Coleman, D. C. 1985. Interactions of bacteria, fungi, and their nematode grazers: Effects on nutrient cycling and plant growth. Ecol. Monogr. 55:119-140.
14. Jaffee, B. A., Tedford, E. C., and Muldoon, A. E. 1993. Tests for density-dependent parasitism of nematodes by nematode-trapping and endoparasitic fungi. Biol. Control 3:329-336.
15. Marshall, A. J. 2002. Sunn hemp (Crotalaria juncea) as an organic amendment in crop production. M.S. thesis, University of Florida, Gainesville.
16. McSorley, R., and Gallaher, R. N. 1995. Cultural practices improve crop tolerance to nematodes. Nematropica 25:53-60.
17. Neher, D. 2001. Role of nematodes in soil health and their use as indicators. J. Nematol. 33:161-168.
18. Okada, H., and Kadota, I. 2003. Host status of 10 fungal isolates for two nematode species, Filenchus misellus and Aphelenchus avenae. Soil Biol. Biochem. 35:1601-1607.
19. van den Boogert, P. H. J. F., Velvis, H., Ettema, C. H., and Bouwman, L. A. 1994. The role of organic matter in the population dynamics of the endoparasitic nematophagous fungus, Drechmaria coniospora in microcrosms. Nematologica 7:139-145.
20. Wang, K.-H., McSorley, R., and Gallaher, R. N. 2003. Effect of Crotalaria juncea amendment in soil with different agricultural histories. J. Nematol. 35:294-301.
21. Wang, K.-H., R. McSorley, and R. N. Gallaher. 2004. Effects of Crotalaria juncea amendment on squash infected with Meloidogyne incognita. J. Nematol. 36:290-296.
22. Wang, K.-H., R. McSorley, and R. N. Gallaher. 2004. Relationships of nematode communities and soil nutrients in cultivated soils. Soil Crop Sci. Soc. Florida Proc. 63:105-113.
23. Wang, K.-H., R. McSorley, A. J. Marshall, and R. N. Gallaher. 2004. Nematode community changes associated with decomposition of Crotalaria juncea amendment in litterbags. Appl. Soil Ecol. 27:31-45.
24. Wang, K.-H., B. S. Sipes, and D. P. Schmitt. 2001. Suppression of Rotylenchulus reniformis by Crotalaria juncea, Brassica napus, and Target erecta. Nematropica 31:237-251.
25. Wang, K.-H., B. S. Sipes, and D. P. Schmitt. 2002. Crotalaria juncea as a cover crop for nematode management: A review. Nematropica 32:35-57.
26. Wasilewska, L., and P. Bienkowski. 1985. Experimental study on the occurrence and activity of soil nematodes in decomposition of plant material. Pedobiologia 28:41-57.
27. Yeates, G. W., T. Bongers, R. G. M. DeGoede, D. W. Freckman, and S. S. Georgieva. 1993. Feeding habits in soil nematode families and genera-An outline for soil ecologists. J. Nematol. 25:101-313.
28. Yeates, G. W., and D. A. Wardle. 1996. Nematodes as predators and prey: relationships to biological control and soil processes. Pedobiologia 40:43-50.