Fusarium head blight (FHB) or scab
Fusarium graminearum (anamorph)
Gibberella zeae (teleomorph)
Note: Several species of Fusarium cause symptoms of Fusarium head blight in wheat. These include, but are not limited to, Fusarium avenaceum, Fusarium culmorum, and Fusarium poae. This lesson, however, will focus solely on Fusarium graminearum sensu stricto, the predominant causal agent of FHB in most areas of the world.
Wheat (Triticum aestivum), Durum Wheat (Triticum durum), Barley (Hordeum vulgare) and Oat (Avena sativa). F. graminearum parasitizes roots, stems, leaves, and reproductive tissues of many species of cereals and grasses.
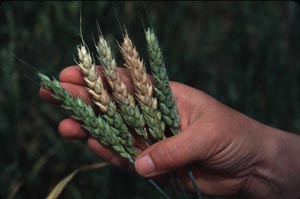
Fusarium head blight in wheat.
Fusarium head blight (FHB), caused by the fungal plant pathogen Fusarium graminearum (Gibberella zeae), is a devastating disease of wheat and barley. Diseased spikelets exhibit symptoms of premature bleaching shortly after infection. The fungus produces a mycotoxin known as deoxynivalenol that poses a significant threat to the health of domestic animals and humans. Disease forecasting models may help to optimize FHB management by targeting fungicide and biocontrol applications.
ACKNOWLEDGEMENTS: The authors thank C. Griffey, M. Keller, and M. McMullen for their helpful and constructive comments during the update of this lesson in 2010.
Symptoms and signs
Individual plants of cereal crops (e.g. wheat) produce multiple stems, and each stem produces a single seed spike which emerges at the end of the stem. The spike is composed of multiple spikelets positioned on alternate sides of the spike’s stem. Each spikelet is composed of flowering structures where seed develops. The first symptoms of Fusarium head blight occur shortly after flowering. Diseased spikelets exhibit premature bleaching as the pathogen grows and spreads within the head (Figure 2). One or more spikelets located in the top, middle, or bottom of the head may be bleached (Figure 3). Over time, the premature bleaching of the spikelets may progress throughout the entire head.
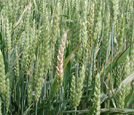 Figure 2 |
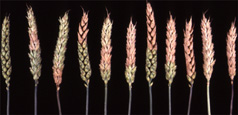 Figure 3 |
If the environment is warm and moist, aggregations of light pink/salmon colored spores (sporodochia) may appear on the rachis and glumes of individual spikelets (Figure 4).
Later in the season, bluish- black spherical bodies may appear on the surface of affected spikelets. These bodies are sexual structures of the fungus known as perithecia, and can be seen readily in laboratory cultures on carrot agar medium (Figure 5).
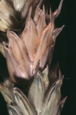 Figure 4 |
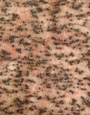 Figure 5 |
As symptoms progress, the fungus colonizes the developing grain causing it to shrink and wrinkle inside the head (Figure 6). Often, the infected kernels have a rough, shriveled appearance, ranging in color from pink, soft-gray, to light-brown (Figure 7).
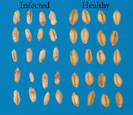 Figure 6 |
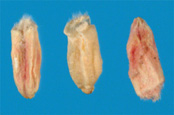 Figure 7 |
Pathogen Biology
Fusarium graminearum is an ascomycete, producing sexual spores in a sac known as an ascus (plural asci). The asexual stage of the fungus produces spores called macroconidia, and the sexual stage produces spores called ascospores.
Asexual reproduction
The anamorph (asexual stage) of the fungus causing FHB is Fusarium graminearum. Macroconidia (asexual spores) are derived from conidium-producing cells called phialides (Figure 8). The phialides are clustered together in cushion-shaped masses known as sporodochia. The macroconidia are hyaline, canoe-shaped spores usually with five or more septa.
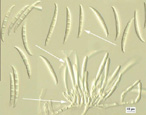 Figure 8
|
Sexual reproduction
The teleomorph (sexual stage) of the fungus is Gibberella zeae. The genus Gibberella belongs in the Hypocreaceae, a family chracterized by brightly colored perithecia that frequently form in stromata (somatic structures on which fruiting bodies develop). The perithecia of G. zeae are a dark blue in color at maturity (Figure 9). Ascospores (sexual spores) form within sacs called asci, and are forcibly discharged from the perithecium through a single small opening known as an ostiole (Figure 10). The ascospores are hyaline to light brown in color, and slightly curved with rounded ends (Figure 11).
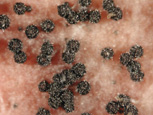 Figure 9 |
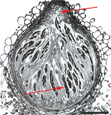 Figure 10 |
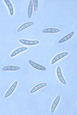 Figure11 |
The majority of the isolates of F. graminearum are homothallic, meaning that they are able to sexually reproduce without a partner. Heterothallic isolates, which require a compatible partner for sexual reproduction, are less common. Laboratory studies, however, have demonstrated that many of the homothallic isolates have the ability to outcross with other compatible isolates. The degree to which this occurs in the field under natural conditions is unclear.
Mycotoxins
Many Fusarium species (including F. graminearum) produce mycotoxins-- fungal chemicals that are harmful to animals. These chemicals may operate in nature to disable plant defense mechanisms or to defend the fungus against other microorganisms. The major toxin produced by F. graminearum in association with FHB in wheat and barley is deoxynivalenol (DON). DON is sometimes called vomitoxin because of its deleterious effects on the digestive system of swine and other monogastric animals. DON disrupts normal cell function by inhibiting protein synthesis. Humans consuming flour made from wheat contaminated with DON will often demonstrate symptoms of nausea, fever, headaches, and vomiting.
DON contamination is measured in parts per million (ppm). DON levels in FHB-infected wheat are frequently quite high (>20 ppm). The USDA recommends that DON levels in human foods not exceed 1 ppm. However, individual grain buyers may have lower tolerances of DON in purchased grain. The FDA has various guideline levels of DON permissible in livestock feed: ruminant animals, such as feeder cattle, are the most tolerant, while swine have the highest sensitivity to DON in livestock feed, with pigs refusing feed containing 1ppm of DON.
Disease Cycle and Epidemiology
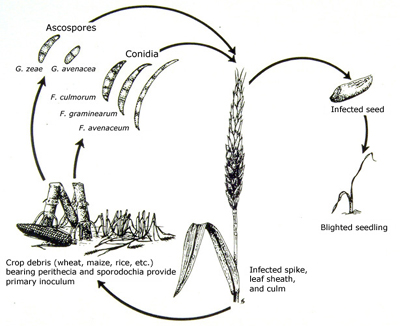 Disease Cycle |
The disease cycle
Fusarium graminearum overwinters on infested crop residues (corn stalks, wheat straw, and other host plants) (Figure 12). On infested residues, the fungus produces asexual spores (macroconidia) which are dispersed to plants and other plant debris by rain-splash or wind. When conditions are warm, humid, and wet, the sexual stage of the fungus (Gibberella zeae) develops on the infested plant debris. Bluish-black perithecia form on the surface of these residues, and forcibly discharge sexual spores (ascospores) into the air (Figure 13). The ascospores are picked up by turbulent wind currents and may travel great distances in the air.
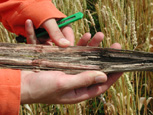 Figure 12 |
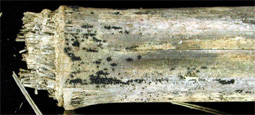 Figure 13 |
Infection occurs when the ascospores (and also macroconidia) land on susceptible wheat heads. Extruded anthers during wheat anthesis (flowering) are thought to be the site of primary infection (Figure 14). If the anthers are infected just after their emergence, the fungus will colonize and kill the florets and kernels will not develop. Florets that are infected later will produce diseased kernels that are shriveled and wilted, or "tombstone" in appearance. Kernels that are colonized by the pathogen during late kernel development may not appear to be affected, but may still be contaminated with the mycotoxin DON (see Pathogen Biology for more information about mycotoxins).
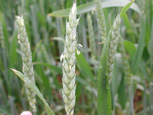 Figure 14 |
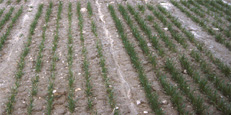 Figure 15 |
Infected kernels may be used as seed for a subsequent wheat crop. These infected seeds, if left untreated, may give rise to blighted seedlings (Figure 15). The degree to which this occurs in the field depends on the percentage of infected seed and soil conditions affecting the growth and development of the seedlings.
The aerobiology of Gibberella zeae
Viable propagules (ascospores and macroconidia) of G. zeae exist in the air before, during, and after wheat flowering. Generally, the majority of spores dispersed from crop residues travel only short distances, but given appropriate weather conditions and wind, spores may spread over long distances.
Using remote- controlled aircraft (Figure 16) and boats equipped with spore-sampling devices (Figure 17) researchers are studying the long-range aerial dispersal of the pathogen. Spores of G. zeae have been collected hundreds of feet in the air over agricultural fields, forests, and lakes.
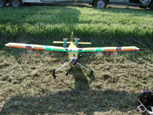 Figure 16 |
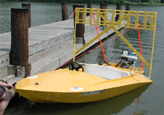 Figure 17 |
There is considerable debate on the relative importance of local vs. long distance spread of inoculum on disease development. In years with highly favorable weather conditions for pathogen spread and infection, local management of overwintered inoculum (e.g., tillage, spraying of debris, etc.) may have negligible impact on disease levels unless performed over extensive production areas.
The environment and FHB
FHB infection is favored by extended periods of high moisture or relative humidity (>90%) and moderately warm temperatures (between 15 to 30°C/ 59 to 86°F). These conditions present before, during, and after flowering favor inoculum production, floret infection, and colonization of developing grains.
FHB is well suited for disease forecasting because of its narrow time periods of pathogen sporulation, spore dispersal, and host infection that contribute to epidemic development. Disease forecasting models for FHB have been developed and are widely used. These models incorporate factors such as temperature, humidity, rainfall, inoculum production, and plant development as predictors for the severity of FHB.
Disease Management of Fusarium head blight
Resistant cultivars
Since 1990, an extensive research endeavor has focused on development and use of resistant cereal cultivars and integrated pest management systems for the control of Fusarium head blight. Thousands of plant lines are subjected to artificial inoculation with F. graminearum (Figure 18). Those lines having reduced fungal growth and low levels of seed contamination with the mycotoxin DON are selected and advanced in additional breeding trials. To date, sources of resistance conferring complete resistance to FHB have not been identified in wheat. Quantitative Trait Loci (QTL) composed of one or more genes, such as Fhb1 derived from the Chinese wheat cultivar Sumai 3, have been identified in wheat. However, these genes confer only partial resistance to FHB, and many of the initial sources of resistance were not well adapted to most of the grain production regions of the U.S. While some success has been made in transferring FHB resistance from such exotic sources into adapted cultivars, identification and deployment of FHB resistance already present in local native germplasm and cultivars is providing another means to achieve this goal. Ultimately, control of FHB, to meet the very low DON limits in wheat grain, will require an integrated approach including development of cultivars having multiple resistance genes and use of fungicides.
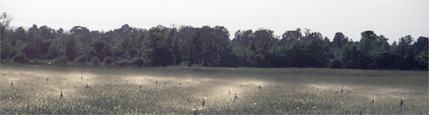 Figure 18 |
Agronomic considerations
Crop sequence (what crops were planted and when) and tillage (soil incorporation of crop residues) have been shown to affect the incidence of FHB. In recent years, decreases in tillage are thought to have contributed to regional scab epidemics by increasing levels of inoculum available for infection.
Since the risk of FHB depends on a viable inoculum source, the management of cereal debris on the soil surface may or may not impact the level of FHB. The relative contribution of inoculum from local and distant sources is not yet fully understood. In regions where there is a significant source of airborne inoculum, local management of the disease (on a single farm) may not be effective.
Fungicides
Chemical controls, such as fungicides, provide partial control of FHB and associated mycotoxin contamination. A number of foliar fungicides have been used to manage FHB in some areas and are applied around the period of wheat flowering. In many areas, fungicides are rarely used for FHB control because of high cost, variable efficacy, and the erratic nature of FHB epidemics. Research continues to identify fungicides that are more effective for the control of FHB.
Many commercial fungicides that are routinely used for cereal seed treatment also reduce the risk of Fusarium seedling blight. Recently (2008) registered fungicides have reduced FHB severity and DON levels in the range of 50-60% across multi-state tests.
Biological Control
Several investigators are focused on finding affordable and environmentally compatible biocontrol agents for the management of FHB. Biocontrol agents could play an important role in organic cereal production. In conventional production, such agents may extend protection of spikes past the flowering stage after fungicides can no longer be applied. Certain strains of spore- producing bacteria (such as Bacillus species) and yeasts (such as Cryptococcus flavescens) show some promise for the control of FHB and the reduction of mycotoxin contamination (Figure 19).
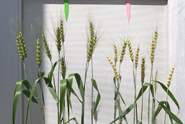 Figure 19 |
Integrated management
Integrated management of FHB may one day be achieved by the combined application of biocontrol agents and fungicides to flowering wheat and barley varieties with partial resistance. Disease forecasting models may help to optimize FHB management by targeting fungicide and biocontrol applications.The online Fusarium Head Blight Risk Assessment Tool (http://www.wheatscab.psu.edu/riskTool_2010.html) may be used to gauge the relative risk of FHB in wheat fields in the U.S. A public outreach program called Scab Smart (http://www.ag.ndsu.edu/scabsmart/) provides U.S. wheat and barley growers with the latest information on integrated management tools that can be applied in their section of the country. Disease Forecasting models help producers determine the risk of FHB infection at the flowering period of wheat, and thus help optimize FHB management by having fungicides applied only when models indicate that the risk of FHB infection is present, based on current weather parameters. The spring wheat model also has the option for the producer to choose the level of resistance present in the wheat variety grown. Level of resistance affects the FHB risk.
Significance
Losses and economic impact
Fusarium head blight is one of the most devastating plant diseases in the world. The United States Department of Agriculture (USDA) ranks FHB as the worst plant disease to hit the US since the rust epidemics in the 1950s. Since 1990, wheat and barley farmers in the United States have lost over $3 billion dollars due to FHB epidemics (Figure 20). Canada has also experienced severe losses since 1990.
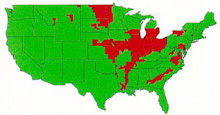 Figure 20 |
|
Mycotoxins as a threat to human health
The mycotoxins produced by F. graminearum may pose a serious threat to human and domestic animal health. Grain that has been infected with the fungus may become incorporated into our staple diets. Strains of the fungus from different countries produce different toxins, some potentially more potent and dangerous than those from strains currently in the United States.
The years ahead
Many fear that the disease will continue to be the cause of major crop losses in the United States and beyond, with the potential to put a major dent in the world's food supply during the years to come. Currently, low commodity prices, increasingly stringent tolerances for mycotoxins, and lack of affordable and highly efficacious control methods, have made wheat and barley production unprofitable in many areas of North America. Who will grow the grain to produce our bread, pastries, pasta, and beer in the years ahead (Figure 21)?
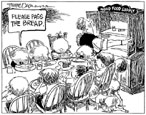 Figure 21 |
The US Wheat and Barley Scab Initiative
The US Wheat and Barley Scab Initiative (USWBSI) was formed to stop Fusarium head blight from further damaging wheat and barley crops in the USA. The USWBSI involves unprecedented cooperative efforts among many federal, state, and private research scientists, as well as growers and associated industries.
Selected References
Bai, G., and G. Shaner. 1994. Scab of wheat: prospects for control. Plant Disease. 78:760-766.
Del Ponte, E.M., J.M.C. Fernandes, and G.C. Bergstrom. 2007. Influence of growth stage on Fusarium head blight and deoxynivalenol production in wheat. Journal of Phytopathology 155:577-581.
De Wolf, E.D., L.V. Madden, and P.E. Lipps. 2003. Risk assessment models for wheat Fusarium head blight epidemics based on within-season weather data. Phytopathology 93:428-435.
Goswami, R.S., and H.C. Kistler. 2004. Heading for disaster: Fusarium graminearum on cereal crops. Molecular Plant Pathology 5:515-525.
Leonard, K.J., and W. Bushnell. 2003. Fusarium Head Blight of Wheat and Barley. APS Press, St. Paul, MN.
McMullen, M., S. Halley, B. Schatz, S. Meyer, J. Jordahl, and J. Ransom. 2008. Integrated strategies for Fusarium head blight management in the United States. Cereal Research Communications 36:563-568.
McMullen, M.P., R. Jones, and D. Gallenberg. 1997. Scab of wheat and barley: a re-emerging disease of devastating impact. Plant Disease. 81:1340-1348.
Nganje, W.E., D.A. Bangsund, F.L. Leistritz, W.W. Wilson, and N.M. Tiapo. 2004. Regional economic impacts of Fusarium head blight in wheat and barley. Review of Agricultural Economics 26:332-347.
Pestka, J.J., and A.T. Smolinski. 2005. Deoxynivalenol: Toxicology and potential effects on humans. Journal of Toxicology and Environmental Health Part B: Critical Reviews 8:39-69.
Schmale, D.G., and G.P. Munkvold. 2009. Mycotoxins in crops: A threat to human and domestic animal health. The Plant Health Instructor. DOI: 10.1094/PHI-I-2009-0715-01.
Windels, C.E. 2000. Economic and social impacts of Fusarium head blight: changing farms and rural communities in the Northern Great Plains. Phytopathology 90:17-21.
WWW Links
Return of an old problem: Fusarium head blight of small grains
http://admin.apsnet.org/publications/apsnetfeatures/Pages/HeadBlight.aspx
Fusarium head blight in Canada
http://www.grainscanada.gc.ca/information/fhb-e.htm
United States Wheat and Barley Scab Initiative
http://scabusa.org/
Fusarium Head Blight Risk Assessment Tool
http://www.wheatscab.psu.edu/riskTool_2010.html
Scab Smart
http://www.ag.ndsu.edu/scabsmart/