Disease: Rhizomania
Pathogen: Beet necrotic yellows virus (BNYVV)
Host: Sugar beet
Introduction
Figure 1. Cystosori of
Polymyxa betae within sugar beet rootlet tissue.
|
Rhizomania is a soilborne root rot disease, which is very unusual for a disease caused by a virus. Furthermore, the viral pathogen is uniquely transmitted to host roots by an obligate, fungal-like, plasmodiophorid parasite, named
Polymyxa betae (Rush 2003, 2009). This organism is ubiquitously distributed worldwide in fields wherever sugar beets have a history of cultivation. It has a restricted host range consisting of members in the
Chenopodiaceae,
Aizoaceae, and
Amaranthaceae families, including spinach, Swiss chard, and several weed species such as pigweed, lambsquarters, and Kochia (Harveson 2007; Harveson et al. 2013). Without this soil-inhabiting parasite, the disease cannot occur; however,
P. betae does not always contain the virus. The vector itself is essentially harmless, causing little damage unless it is carrying the virus and transmits it to the host plant's roots after infection (Harveson 2008; Rush and Heidel 1995). Its presence can be readily confirmed by observing the clusters of thick-walled cystosori within roots in wet mounts under a compound microscope (Fig. 1).
Symptoms and Signs
Foliar symptoms of rhizomania within production fields consist of wilting (Fig. 2) and a general chlorosis of foliage. Affected plants commonly exhibit varying degrees of yellowing of foliage and often have an erect, upright posture (Fig. 3). Because infected roots take up water inefficiently, foliar symptoms may resemble several abiotic stresses, such as water or nitrogen deficiencies. The chlorosis and wilting of foliage may also be confused with other root diseases, such as Aphanomyces root rot (Fig. 4) or Fusarium yellows (Fig. 5), but without the interveinal yellowing or leaf scorching symptoms also characteristic of these diseases (Fig. 6) (Harveson, 2006). Systemic infection is uncommon, but if it occurs, foliar symptoms consist of yellow vein clearing (Fig. 7), which may later turn necrotic (Fig. 8). This seldom-seen symptom is the source of the pathogen's name—
beet necrotic yellow vein virus.
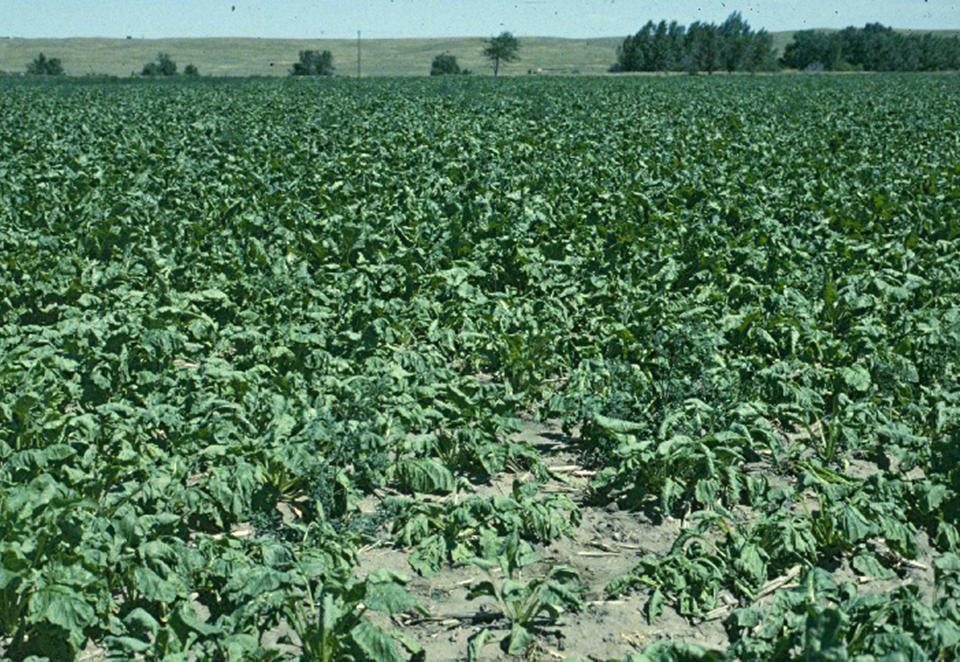 Figure 2. Wilting symptoms characteristic of rhizomania in a sugar beet production field.
| Figure 3. Infected chlorotic sugar beet plant with abnormally upright growth.
| Figure 4. Foliar symptoms of Aphanomyces root rot caused by
A. cochlioides.
| Figure 5. Foliar symptoms of Fusarium yellows symptoms caused by
F. oxysporum f. sp.
betae.
|
Figure 6. Wilting and leaf scorching characteristic of both Aphanomyces root rot and Fusarium yellows.
| Figure 7. Vein clearing symptoms of rhizomania characteristic of systemic infection.
| Figure 8. Vein clearing symptoms becoming necrotic. It is the source of pathogen's name, beet necrotic yellow vein virus.
| Figure 9. Tan to light brown vascular tissues characteristic of beet necrotic yellow vein virus or early Fusarium oxysporum f. sp. betae infections.
|
Figure 10. Bearding symptoms with secondary rootlets and stunted tap roots, characteristic of early root infections and source of the disease name “rhizomania" ("root madness").
| Figure 11. “Wineglass" symptoms characteristic of later root infections.
| | |
Disease severity and economic losses are usually dependent on time of infection (Rush 2009). Root symptoms begin as a light brown discoloration of the central stele within the taproot. Infections by
Fusarium oxysporum f. sp. betae (causal agent of Fusarium yellows) also cause similar symptoms (Fig. 9), so diagnostic tests may be required to distinguish between the two pathogens and diseases (Harveson 2006; Rush 2009). Classical root symptoms following early infection include small, severely stunted taproots with masses of fine, hairy secondary rootlets, giving the roots a “bearded" appearance (Fig. 10). This symptom is the origin of the name “rhizomania," meaning “root madness" or “crazy root" (Biancardi et al. 2002; Franc et al. 1993). Infections occurring later in the season can appear healthy aboveground, but often cause roots to become rotted and/or constricted, resulting in a wineglass-shaped taproot (Fig. 11) (Harveson 2008; Rush 2003; Rush and Heidel 1995).
Pathogen Biology
BNYVV is the causal agent of the disease rhizomania, but it survives in and is transmitted by the soilborne plasmodiophorid parasite,
P. betae. BNYVV is the type member and a species of the viral genus Benyvirus (Rush 2003, 2006). Wild-type isolates generally possess multipartite genomes consisting of four to five rigid, tubular rod particles with positive-sense single-stranded RNA. These particles are all approximately 20 nm in diameter, with differing lengths ranging from 80 to 390 nm (Acharya et al. 2017; Rush and Heidel 1995).
Pathogen isolates usually contain four RNA particles, with some European isolates having a fifth RNA particle (Acharya et al. 2017). RNAs 1 and 2 are required for virus infections. RNA 3 encodes amplification in roots from field infections and controls symptom development and expression with the production of the proliferous rootlets (“bearding"). RNA 4 is required for adequate transmission of the pathogen by the vector, P. betae. RNA 5 is not always present, but those isolates carrying it are more virulent compared to those possessing only RNAs 1–4 (Acharya et al. 2017; Galein et al. 2018; McGrann et al. 2009). Furthermore, RNA 5 appears to be involved inexpediting pathogen movement systemically within the root system (Rush and Heidel 1995).
Additionally, several distinct strains of the virus have been identified, including A, B, and P types. A and B types are the two most commonly found types. The A type is more prevalent worldwide (in most of Europe, North America, Japan, and China), while the B type is somewhat restricted, being more common in France, Germany, and Great Britain (Acharya et al. 2017; McGrann et al. 2009). Only a few P type strains have been found in France, the United Kingdom, and Kazakhstan, but they are more aggressive and contain the additional RNA 5. The P type strains reach higher concentrations of the pathogen within the vector and also move more rapidly within plants, avoiding plant defense responses. Thus, these strains are considered to be more of a threat to the sugar beet industry worldwide if control measures were based exclusively on genetics or disease resistance (Acharya et al. 2017; McGrann et al. 2009).
Disease Cycle and Epidemiology
Despite other chenopodiaceous plants being susceptible, sugar beets serve as the primary economic host for both the parasitic vector and the viral pathogen. Some weeds can also harbor these organisms, but their role in the development and spread of the disease is unclear (Harveson 2007).
The
P. betae vector produces two types of spores during its life cycle, including resting spores and motile tadpole-like zoospores (Acharya et al. 2017; Rush 2009; Teakle 1983). P. betae survives in soil or root debris as thick-walled, long-lived structures called cystosori (resting spores), and the pathogen survives within these structures (Teakle 1983). These resting spores enable both the parasite and viral pathogen to survive in soil for many years in the absence of a host. When a host is present under soil conditions of high moisture and warm temperatures, the cystosori germinate, releasing viruliferous zoospores. After locating and attaching to hosts, zoospores encyst and penetrate into cells, injecting their cytoplasm containing particles of the viral pathogen into plants (Acharya et al. 2017; McGrann et al. 2009; Rush et al. 2006).
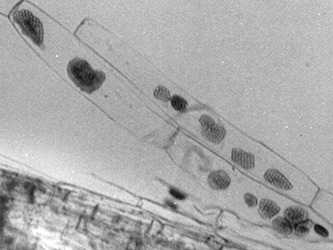 Figure 12. Clusters of cystosori of beet necrotic yellow vein virus vector,
Polymyxa betae, in epidermal cells of infested small sugar beet rootlets. These tissues slough off in the soil as roots decay and is the manner in which the vector survives.
|
The host will try to compensate for the infection with the production of new rootlets, producing the “bearded" root symptom in the plants (Harveson 2006; Harveson et al. 2013). Once inside the host,
P. betae develops new plasmodia and zoosporangia, resulting in additional flushes of secondary zoospores that are released and attracted to newly formed rootlets when soil conditions are favorable for infection (McGrann et al. 2009; Rush 2003). This repeating infection life cycle requires approximately 48 h to complete, which subsequently rapidly increases both the parasite and pathogen. The overwintering resting spores formed in roots can then be released back into soils as tissues degrade at the end of the season (Fig. 12) (Harveson 2008).
Root infection is favored by relatively high soil temperatures, with an optimum of 23 to 27°C. Infections are sharply reduced in the presence of cooler temperatures, requiring a minimum of approximately 15°C for germination of resting spores (Rush 2003, 2009). Therefore, warm soil temperatures in the spring and early summer can result in earlier infections, causing greater degrees of damage to the young plants (Harveson et al. 2013). Because zoospores require free moisture for movement, soil moisture at or near saturation for prolonged periods is necessary for infection and disease development. Soil pH and texture may also play roles in disease development, with neutral to slightly alkaline (pH 6–8) and coarse-textured soils favoring disease development (Franc et al. 1993; Harveson et al. 2013; Rush 2009).
Infections and the appearance of diseased, symptomatic plants tend to occur in clustered patches in the field and not as scattered individual plants dispersed randomly throughout the field. Because the parasite vector thrives in wet areas, disease incidence and severity are usually greatest in poorly drained portions of the field. Reduced water uptake by infected roots increases the tendency for soil around diseased plants to remain waterlogged, enhancing and promoting additional disease development of BNYVV accompanied byroot rotting by various saprophytic, opportunistic microorganisms (Rush 2003; Rush et al. 2006).
Management
Chemical: No fungicides are available that have any activity for managing the
Polymyxa vector. The only chemicals ever proven to successfully manage rhizomania have been fumigants. Studies in the United States have attained significant decreases in disease severity and incidence with improved yields after fumigating soils in sugar beet fields with methyl bromide, 1,3-dichloropropene (Telone), or metam-sodium (Harveson and Rush 1994; Martin and Whitney 1990). However, fumigation can be difficult, dangerous, and cost-prohibitive, as well as not always being a sustainable option.
Cultural Practices: A combination of cultural practices aimed at interfering with the vector's life cycle will help minimize severe infections and yield reductions. Early planting, when soil temperatures are cooler, and production practices that result in the rapid establishment of the plant canopy will reduce risk of loss. Managing soil moisture during the first 6 weeks following seed germination by avoiding over-irrigation or any other practices that result in standing water or excessively wet soil are also important. Proper fertility practices must be followed to reduce plant stresses and further reduce the risk of disease development. Runoff water from infested fields should be contained to prevent possible movement of viruliferous spores to downstream sites; however, it has also been demonstrated previously that physical movement of infested soils during tillage and harvest operations was more influential on the spread of BNYVV than any type of water events (Harveson and Rush 1996; Tuitert 1993). Deep tillage to improve drainage or sanitation of farm equipment before entering new fields also helps reduce disease risk. In general, due to the dependence of the pathogen on its vector, any factor that prohibits infection by
P. betae and its spread within or among fields will directly limit or reduce the incidence and severity of rhizomania.
Genetic Resistance: Due to the lack of safe, dependable chemical options for disease management, the use of resistant cultivars has been considered the most effective and least expensive tool for managing this disease, and successful disease resistance breeding has greatly reduced damage in sugar beets. Fortunately, resistance to the pathogen was identified early and incorporated into new cultivars, resulting in successful production in problem areas highly infested with the pathogen.
The search for disease resistance began in 1970s, but the first commercially accepted sources were not developed for another 10 years (McGrann et al. 2009). The first type of resistance recognized was called “Type Alba" and was obtained from a
Beta germplasm collection of the Italian seed company Alba. These first efforts with Alba-type resistance were positive, but a large step forward occurred with a more resistant variety, “Rizor," released in 1985 in Italy by Marco Di Biaggi (Stevanato and Panella 2013). It was determined that the viral pathogen's movement throughout roots was hindered by Rizor-type resistance compared to susceptible genotypes, and this type of resistance was recognized as monogenic and dominant.
Other additional hybrids using this gene soon were developed that surpassed Rizor in agronomic characteristics, but in 1986 further progress was achieved using another gene discovered and developed in California, also with single gene, dominant resistance. The so-called “Holly" gene was first identified by A. W. Erichsen at Tracy, CA, with the Holly Sugar Company, and regressed into new cultivars by Robert Lewellen with the USDA-ARS in Salinas, CA (Lewellen et al. 1987; Stevanato and Panella 2013). It conferred strong resistance against BNYVV and was named Rz1. This gene has now been used worldwide in the production of many new commercially available cultivars. Plants possessing Rz1 are still susceptible, but virus titers (concentrations) remain low, and infected plants normally are not symptomatic and grow normally. Both the Holly and Rizor resistance genes appear to limit the spread and replication rate of the virus within infected roots (Stevanato and Panella 2013). A second, monogenic resistance gene, distinct from Rizor/Holly, was identified in 1987 by the breeding program of Robert Lewellen from a population of sea beets originating in Denmark and called Rz2 and is widely used now (Galein et al. 1987; Lewellen et al. 1987; Liu and Lewellen 2007).
Figure 13. Foliar symptoms of rhizomania infections (bright yellow upright growth) in the field occurring in a disease tolerant cultivar referred to as
“Blinkers."
|
During the 2002–2003 growing season, sugar beets in the Imperial Valley of California planted with disease tolerant cultivars began expressing symptoms consistent with rhizomania. The occurrence of the disease was observed in large field-length strips from multiple fields, and some of the isolates from these affected plants were verified to have overcome the genetic resistance of Rz1 (Liu et al. 2005; Rush et al. 2006). A similar phenomenon occurred during that same season in fields in southern Minnesota sown with rhizomania-tolerant cultivars. However, the distribution of the symptoms was different from those in California. They occurred in clusters within fields producing individual plants showing chlorotic symptoms. These symptomatic plants were referred to as “blinkers" (Fig. 13) and were also confirmed to be affected by BNYVV, indicating that resistance to Rz1 had been compromised.
To date, additional resistance-breaking isolates of BNYVV in the United States have been discovered in soil sample assays from several other growing areas (Liu and Lewellen 2007), but no evidence for the loss of the Rz2 resistance gene has been demonstrated. The appearance of resistance-breaking strains in some locations is presumed to be a result of the former practice of continual reliance on the single gene (Rz1) in resistant cultivars, encouraging the selection of isolates capable of overcoming resistance to BNYVV (McGrann 2009). New improved cultivars containing both Rz1 and Rz2 are now routinely being produced to inhibit or slow the emergence of additional resistance-breaking BNYVV strains.
Significance
The first generation of rhizomania-resistant cultivars did well in highly infested fields, but in fields with low levels of BNYVV, yields were inferior to virus-susceptible cultivars. Breeders rapidly improved agronomic traits in new cultivars also containing disease resistance. The use of genetic resistance and highly tolerant or resistant cultivars has been extremely effective but has not completely eradicated the disease. However, it has allowed yields in areas previously damaged severely by epidemics to remain at levels adequate for continued production. (Biancardi et al. 2002). Nevertheless, each variety must be tested regionally to evaluate performance under local environmental conditions and production practices. In fact, for the Western Sugar Cooperative growing areas of the Central High Plains, it is mandatory for each new cultivar to possess resistance to rhizomania before they are approved for use in Colorado, Nebraska, Montana, and Wyoming.
Because both the vector and pathogen are capable of surviving in soils for years, if not decades, this pathogen remains a constant tenant in soils once introduced, or it may overcome genetic resistance, as has occurred in several growing areas of the United States. Single gene resistance enabled breeders to rapidly create new cultivars, but it also created better opportunities for the pathogen to overcome resistance. The appearance of new resistant-breaking isolates of BNYVV poses some concern. Although they appear to be localized in specific areas and are not yet widespread, they still pose a potential risk, and their appearance has accelerated the search for additional resistant sources in the continuing struggle with this disease.
References
Acharya, L., Hota, S., and Pramanik, K. 2017. Rhizomania – a review. Int. J. Curr. Microbiol. App. Sci. 6: 358-367.
Biancardi, E., Lewellen, R. T., De Biaggi, M., Erichsen, A. W., and Stevanato, P. 2002. The origin of rhizomania resistance in sugar beet. Euphytica 127: 383-397.
Dufus, J. E., Whitney, E. D., Larse, R. C., Liu, H.-Y., and Lewellen, R. T. 1984. First report in the western hemisphere of rhizomania of sugar beet caused by beet necrotic yellow vein virus. Plant Dis. 68: 251.
Franc, G. D., Kerr, E. D., Brown, W. M., and Riesselman, J. H. 1993. Rhizomania of sugar beet. In cooperation with Colorado State University, Montana State University, University of Nebraska, and USDA-ARS. Published by University of Wyoming, Cooperative Extension Service, B-979.
Galein, Y., Legreve, A., and Bragard, C. 2018. Long term management of rhizomania disease – insight into the changes in beet necrotic yellow vein virus RNE-3 observed under resistant and non-resistant sugar beet fields. Front. Pl. Sci. 9: 795.
Harveson, R. M., and Rush, C. M. 1994. Evaluation of fumigation and rhizomania-tolerant cultivars for control of a root disease complex of sugar beets. Plant Dis. 78: 1197-1202.
Harveson, R. M., and Rush, C. M., and Wheeler, T. A. 1996. The spread of beet necrotic yellow vein virus from point source inoculations as influenced by irrigation and tillage. Phytopathology 86: 1242-1247.
Harveson, R. M. 2006. Identifying and distinguishing seedling and root rot diseases of sugar beets. Online. Plant Health Progress, doi: 10.1094/PHP-2006-0915-01-DG.
Harveson, R. M. 2007. The Relationship of Dry Bean and Sugar Beet Pathogens with Common Weeds in Nebraska Production Fields. NebGuide G1678.
Harveson, R. M. 2008. Rhizomania of sugar beet. NebGuide G1842.
Harveson, R. M., Jacobsen, B., Franc, G. D., and Kerr, E. D. 2013. Disease management. Pages 157-188 in: Sugar Beet Production Guide (revised). R. G. Wilson, J. A. Smith, eds. In cooperation with Colorado State University, Montana State University, University of Wyoming, and USDA-ARS. Published by University of Nebraska, Cooperative Extension, EC01-156. 244 pp.
Lewellen, R. T., Skoyen, I. O., and Erichsen, A. W. 1987. Breeding sugarbeet for resistance to rhizomania: evaluation of host-plant reactions and selections for an inheritance of resistance. In: Proceedings of the 50th Winter Congress of the IIRB. International Institute for Beet research, Brussels, Belgium, pp.136-156.
Liu, H.-Y., Sears, J. L., and Lewellen, R. T. 2005. Occurrence of resistance-breaking Beet necrotic yellow vein virus of sugar beet. Plant Dis. 89: 464-468.
Liu, H.-Y., and Lewellen, R. T. 2007. Distribution and molecular characterization of resistance-breaking isolates of beet necrotic yellow vein virus in the United States. Plant Dis. 91: 847-851.
Martin, F. N., and Whitney, E. D. 1990. In-bed fumigation for control of rhizomania of sugar-beet. Plant Dis. 74: 31-35.
McGrann, G. R. D., Grimmer, M. K., and Mutasa-Gottgens, E. S. 2009. Progress toward understanding and control of sugar beet rhizomania disease. Mol. Pl. Pathol. 10: 129-141.
Rush, C. M., and Heidel, G. 1995. Furovirus diseases of sugar beets in the United States. Plant Dis. 79: 868-875.
Rush, C. M. 2003. Ecology and epidemiology of Benyviruses and plasmodiophorid vectors. Ann. Rev. Phytopathol. 41: 567-592.
Rush, C. M. 2009. Viruses transmitted by Polymyxa betae. Pages 41-46 in: Compendium of Beet Diseases and Pests. R. M. Harveson, L. E. Hanson, and G. L. Hein, eds. APS Press, St. Paul, MN.
Rush, C. M., Liu, H.-Y., Lewellen, R. T., and Acosta-Leal, R. 2006. The continuing saga of rhizomania of sugar beet. Plant Dis. 90: 4-15.
Stevanato, P., and Panella, L. W. 2013. History of sugarbeets. SugarProducer, February, 2013.
Teakle, D. S. 1983. Zoosporic fungi and viruses – double trouble. Pages 233-248 in: Zoosporic plant pathogens, a modern perspective. S. T., Buczacki, editor. Academic Press, New York.
Tuitert, G. (1993). Horizontal spread of beet necrotic yellow vein virus in soil. Neth. J. Plant Pathol. 99: 85-96.