Sudden death syndrome of soybean
North America: Fusarium virguliforme (Aoki et al., 2003). Synonym: F. solani (Mart.) Sacc. f. sp. glycines; South America: F. brasiliense, F. cuneirostrum, F. tucumaniae , and F. virguliforme (Aoki et al., 2005).
soybean (Glycine max)
Sudden death syndrome (SDS) of soybean was first discovered in 1971 in Arkansas and since then has been confirmed throughout most soybean-growing areas of the U.S. SDS is a fungal disease that also occurs in a disease complex with the soybean cyst nematode (SCN, Heterodera glycines). SDS is among the most devastating soil-borne diseases of soybean in the USA. When this disease occurs in the presence of SCN disease symptoms occur earlier and are more severe. Disease symptoms are most pronounced after flowering.
Symptoms and Signs
Sudden death syndrome (SDS) of soybean is typically not detectable on the foliage of plants until after the beginning of flowering. Under rare circumstances younger plants may show symptoms. It is always useful to compare the affected plants with healthy plants of the same field when making disease assessments.
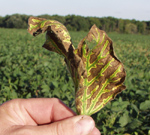 Figure 1a |
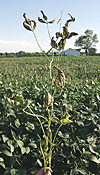 Figure 1b |
 Figure 1c |
Foliage and stems
Early symptoms of SDS are diffuse chlorotic mottling and crinkling of the leaves. Later, leaf tissue between the major veins turns yellow, then dies and turns brown. This interveinal chlorosis and necrosis is typical for SDS, but these symptoms are not diagnostic by themselves because they can be confused with foliar symptoms of other diseases. Soon after, the leaflets die and shrivel. In severe cases, the leaflets will drop off, leaving the petioles attached. For diagnosis, the lower stem and the taproot must be split. The cortical tissue of a plant with SDS will exhibit tan to light brown streaks, whereas the cortex of a healthy plant will be white. The pith (the central portion of the stem) of an infected plant will remain white or slightly cream-colored.
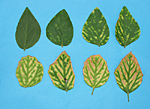 Figure 2a |
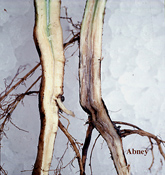 Figure 2b |
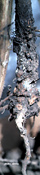 Figure 2c |
Roots
If a plant with advanced foliar symptoms of SDS is removed from the soil, root systems will lack vigor when compared to a healthy root system. Roots may also be rotted. If the plants are collected when soil is moist, small, light-blue patches may be visible on the surface of the taproot near the soil line. These patches are blue spore masses of the fungi that cause SDS. As the root surface dries, the blue color will fade, but these blue spore masses, seen in conjunction with the other symptoms mentioned above, are strong diagnostic indicators of SDS.
Distinguishing SDS from similar diseases
Brown stem rot, stem canker, charcoal rot, and chemical burn can all produce foliar symptoms similar to SDS. However, in these other diseases, dead leaflets tend to remain attached to the petioles. Brown stem rot symptoms differ from SDS symptoms by the darkened pith and insignificant discoloration of the cortex. Stem canker is often associated with spore mats within the stem that are visible as longitudinal, net-like striations. Charcoal rot gets its name from the microsclerotia that develop within stem tissue and that resemble a fine layer of charcoal dust. No signs of pathogens are found when chemical burn is responsible for foliar symptoms and roots of affected plants would most likely appear healthy.
Pathogen Biology
SDS is caused by soil-borne fungi within a group (clade 2) of the Fusarium solani species complex (Aoki et al., 2003; Aoki et al., 2005). In North America, Fusarium virguliforme (Aoki et al., 2003), formerly Fusarium solani f. sp. glycines (Roy, 1997), is the causal agent. In South America, F. brasiliense, F. cuneirostrum, F. tucumaniae, and F. virguliforme cause SDS symptoms. In greenhouse inoculation experiments, F. cuneirostrum and F. phaseoli, causal agents of dry bean root rot, are also able to cause SDS symptoms on soybean (Aoki et al., 2005). Fungi of this taxonomic complex grow slowly in culture and are difficult to isolate from diseased plants. Once a pure culture is obtained, blue spores and other cultural characteristics distinguish the SDS pathogens from other Fusarium species that can infect soybean roots. In North America, the SDS-pathogen is considered clonal and has been considered asexual, but recent crossing experiments revealed the existence of a teleomorph (Covert et al. 2007). One of the South American SDS pathogens, F. tucumaniae, formed fertile perithecia (sexual reproductive structures) in specific crosses under laboratory conditions. Based on the Vienna Code of the International Code of Botanical Nomenclature (Article 59.7) the anamorph name has been retained for this fungus (Covert et al., 2007). Fusarium virguliforme, the North America SDS-pathogen, did not form fertile crosses with itself or with F. tucumaniae; only infertile sexual structures were produced in some of the latter crosses (Covert et al., 2007). These findings support the hypothesis that the North America SDS pathogen is clonal and that distinct species cause SDS in North and South America, respectively.
Presumably, the SDS pathogen survives between soybean crops as chlamydospores in crop residue or freely in the soil (Figure 3a). The thick-walled chlamydospores develop in the soil and on soybean roots during disease development and thereafter. Chlamydospores can withstand wide fluctuations in soil temperature, including freezing, and resist desiccation. As soil warms in the spring, chlamydospores near soybean roots are stimulated to germinate, and then infect soybean roots.
Although the pathogen may produce spores (macroconidia, Figure 3b) on the surface of the taproot during the summer, these spores spread only short distances within a growing season. Over a period of years, flowing water and cultivation practices that move soil can move spores over longer distances within or between fields.
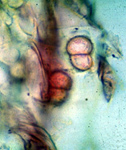 Figure 3a |
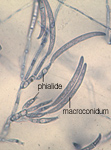 Figure 3b |
Disease Cycle and Epidemiology
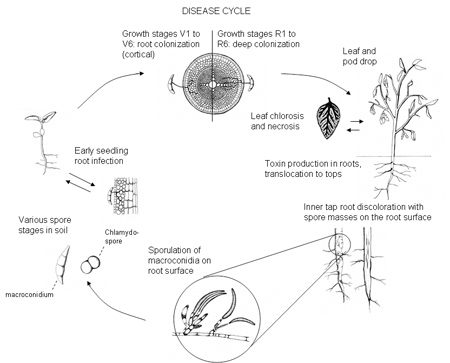
Disease Cycle and Epidemiology
The fungus may infect roots of soybean seedlings soon after planting, but above ground symptoms of SDS in the midwestern USA rarely appear until mid-July, when soybean plants have reached reproductive stages. At this later time, the fungus penetrates into the vascular tissue of the plant (Roy et al., 1997). The fungus produces toxins in the roots that are translocated to the leaves. Often, symptoms first appear after heavy rains during reproductive stages; high soil moisture increases the disease severity (Xing and Westphal, 2006). The toxins cause foliar symptoms; the fungus itself does not invade the stems more than a few centimeters above the soil line (Roy et al., 1997).
The first noticeable symptoms of SDS are yellowing and defoliation of upper leaves. When symptoms first appear in a field, they may be confined to a few small areas or strips in the field, often in wetter or compacted areas (Figura 4), such as turn rows. Over the following two or three weeks, affected areas may enlarge and plants in other areas in the field may show symptoms. The extent of yield losses due to SDS depends on the severity and timing of disease expression relative to plant development in regards to yield components. If the disease develops early in the season, flowers and young pods will abort. When the disease develops later, the plant will produce fewer seeds per pod or smaller seeds. The earlier severe disease develops, the more the yield is reduced. Because the SDS fungus can persist in soil for long periods, larger areas of a field will show symptoms of the disease each growing season until most of the field is affected. No inoculum threshold levels of the pathogen have been developed, but the current hypothesis is that SDS will occur as long as the pathogen is present and conditions are conducive for the disease. SDS is more severe when the soybean cyst nematode (SCN, Heterodera glycines) is also present in a field and the cultivar is susceptible to both pathogens (Xing and Westphal, 2006). Both F. virguliforme and SCN are widespread. The close association of the pathogens is also apparent in the fact that the SDS pathogen can be isolated from cysts of SCN (Roy et al., 1997). It is not fully understood what implications this may have for the epidemiology of the disease. Production practices that maintain SCN at low levels may reduce the risk for SDS, but more research is necessary to better understand the nature of the interaction of the two pathogens. A field infested with the SDS pathogen should also be evaluated for SCN, and if SCN is present, a management strategy for both pests should be implemented.
 Figura 4 |
Disease Management
Management options for SDS are limited. Although soybean cultivars that are less susceptible to SDS have been developed, no highly resistant cultivars are available (Njiti et al., 2002). Fungicides applied in furrow during planting or as seed treatments have only limited effects on disease reduction. Fungicides applied to foliage have no effect on SDS suppression, presumably because the fungal infection is restricted to root systems and fungicides typically do not move downward in the plant to reach this site of infection. Several management practices may reduce the risk of SDS damage, although they will not prevent the disease:
Planting Date
Early planting predisposes soybean to infection. In cool, wet soils, young soybean plants are vulnerable to infection by the SDS pathogen. If early spring conditions are favorable for rapid soybean growth, and if saturating rains do not occur during early reproductive stages of growth, the risk for SDS may be less even though the fungus is present in the soil. Fields with no history of SDS should be planted first; fields where SDS has been a problem should be planted last.
Tillage
Compacted soils impede water percolation and restrict root growth. A heavy rain when soybean has reached the reproductive stages will saturate compacted areas, which promotes SDS development. Correcting soil compaction and water permeability problems may reduce the risk for SDS. Soils respond differently to tillage system intensity changes; plowing, chiseling, or similar drastic soil disturbances strongly affect drainage, crop residue position, and the microbial composition of soil (Aon, 2001; Kladivko, 2001). Not surprisingly, reports on effects of tillage on SDS are contradictory. In some soils, no-tillage can be beneficial in reducing the severity of SDS compared to plow or chisel plow tillage (Seyb et al., 2007; Abney, unpublished). In other soils, intensive tillage reduces SDS presumably by maintaining sufficient vertical water movement compared to no-till (Vick et al., 2006). The best drainage and the most root growth-enhancing soil management may be facilitated in various ways — in some instances, this may require intensive tilling in some soil types, but no-till may be more appropriate in other soil types.
Rotation
Crop rotation may reduce the risk for SDS (Rupe et al., 1997), but corn-soybean in yearly rotation, common in the Corn Belt, does not reduce the incidence and severity of SDS (Westphal, unpublished). Severe outbreaks of the disease have occurred even after several years of continuous corn. Crop rotation reduces the inoculum potential of other soybean pathogens, but shifting to annual rotations of corn and soybean (compared to longer rotations that involved small grains and perhaps forages) fails to reduce the risk for SDS. Studies at Purdue University have found that soybean roots are not visually healthier after a rotation with corn compared to continuous soybean (Xing and Westphal, unpublished). When corn is grown in the field, soilborne pathogens may decline to some extent, but not enough to substantially reduce the disease pressure when soybean is grown in the field the following year. Although a two-year rotation may hold SCN population densities below threshold levels when the initial population density is low, such a rotation appears to be too short to reduce risk for SDS.
Resistant Soybean Cultivars
Soybean breeders are striving to develop SDS-resistant cultivars, but progress has been slow. Greenhouse and field methods, employing high rates of artificially produced fungal inoculum on grain sorghum and carefully selected watering regimes were developed (Hartman et al., 1997; De Farrias et al., 2006). While most seed companies have removed highly susceptible cultivars from their inventories, no highly resistant cultivars are available. Because seed companies continually introduce new cultivars and retire older ones, accurate information about the reaction of new cultivars to SDS is essential.
Under these challenging management conditions, planting highly susceptible soybean cultivars into fields with high risk for SDS must be avoided. Field records of when and where SDS and other soilborne diseases occur are essential for management of SDS and other soilborne diseases. Handheld GPS receivers may assist in this strategy, but even simple sketched maps will help record problem areas. Fields severely affected by SDS should be earmarked for later planting and operations to improve water permeability should be considered, including compaction-correcting tillage or tile drainage. Finally, cultivars with some degree of resistance should be planted.
Significance
Sudden death syndrome (SDS) is one of the most important diseases of soybean in North and South America (Aoki et al., 2005; Wrather and Koenning, 2006; Wrather et al., 2003). SDS first occurred in South America in the early 1990s (Roy et al., 1997). In the U.S.A., SDS was first discovered in Arkansas in 1971. The disease has spread extensively since then and now causes most yield losses throughout the North Central Region. SDS is most severe when soybean is planted early into cool, wet soils, when heavy midsummer rains saturate the soil, and when SCN is present.
Acknowledgements
This manuscript is published with the Purdue University Agricultural Research Program number: 2007-18145. The artwork of K. A. Frank (disease cycle) is greatly appreciated.
Selected References
Aoki, T., K. O’Donnell, Y. Homma, and A.R. Lattanzi. 2003. Sudden-death syndrome of soybean is caused by two morphologically and phylogenetically distinct species within the Fusarium solani species complex-F. virguliforme in North America and F. tucumaniae in South America. Mycologia 95:660-684.
Aoki, T., K. O'Donell, and M.M. Scandiani. 2005. Sudden death syndrome of soybean in South America is caused by four species of Fusarium: Fusarium brasiliense sp. nov., F cuneirostrum sp. nov., F. tucumaniae, and F. virguliforme. Mycoscience 46:162-183. Online: doi:10.1007/s10267-005-0235-y.
Aon, M.A., D.E. Sarena, J.L. Burgos, S. Cortassa. 2001. (Micro)biological, chemical and physical properties of soils subjected to conventional or no-tillage management, and assessment of their quality status. Soil and Tillage Research 60:173-186. Online: doi:10.1016/s0167-1987(01)00190-8.
Covert, S.F., T. Aoki, K. O'Donnell, D. Starkey, A. Holliday, D.M. Geiser, F. Cheung, C. Town, A. Strom, J. Juba, M. Scandiani, X.B. Yang. 2007. Sexual reproduction in the soybean sudden death syndrome pathogen Fusarium tucumaniae. Fungal Genetics and Biology 44:799-807. On-line: doi:10.1016/j.fgb.2006.12.009.
De Farias Neto, A., G.L. Hartman, W.L. Pedersen, S. Li, G. Bollero, and B.W. Diers. 2006. Irrigation and inoculation treatments that increase the severity of soybean sudden death syndrome in the field. Crop Science 46:2547-2554. Online: doi: 10.2135/cropsci2006.02.0129.
Hartman, G.L., Y.H. Huang, R.L. Nelson, and G.R. Noel. 1997. Germplasm evaluation of Glycine max for resistance to Fusarium solani, the causal organism of sudden death syndrome. Plant Disease 81:515-518.
Kladivko, E. 2001. Tillage systems and soil ecology. Soil and Tillage Research 61:61-76. Online: doi:10.1016/s0167-1987(01)00179-9.
Njiti, V.N., K. Meksem, M.J. Iqbal, J.E. Johnson, M.A. Kassem, K.F. Zobrist, V.Y. Kilo, and D.A. Lightfoot. 2002. Common loci underlie field resistance to soybean sudden death syndrome in Forrest, Pyramid, Essex, and Douglas. Theoretical and Applied Genetics 104:294-300. Online: doi: 10.1007/s001220100682.
Roy, K.W. 1997. Fusarium solani on soybean roots: nomenclature of the causal agent of sudden death syndrome and identity and relevance of F. solani form B. Plant Disease 81:259-266.
Roy, K.W., D.E. Hershman, J.C. Rupe, and T.S. Abney. 1997. Sudden death syndrome of soybean. Plant Disease 81:1100-1111.
Rupe, J.C., R.T. Robbins, and E.E. Gbur, Jr. 1997. Effect of crop rotation on soil population densities of Fusarium solani and Heterodera glycines and on the development of sudden death syndrome of soybean. Crop Protection 16:575-580.
Seyb, A., L.J. Xing, T.J. Vyn, J. Seo, T.S. Abney, and A. Westphal. 2007. Tillage system effects on sudden death syndrome, Heterodera glycines, and soybean yield in a Mollisol. Phytopathology 97:S106 (abstract).
Vick, C.M., J.P. Bond, S.K. Chong, and J.S. Russin. 2006. Response of soybean sudden death syndrome to tillage and cultivar. Canadian Journal of Plant Pathology 28:77-83.
Wrather, J.A., and S.R. Koenning. 2006. Estimates of disease effects on soybean yields in the United States 2003 to 2005. Journal of Nematology 38:173-180.
Wrather, J.A., S.R. Koenning, and T.R. Anderson. 2003. Effect of diseases on soybean yields in the United States and Ontario (1999-2002). Plant Health Progress. Online: doi:10.1094/PHP-2003-0325-01-RV.
Xing, L.J., and A. Westphal. 2006. Interaction of Fusarium solani f. sp. glycines and Heterodera glycines in sudden death syndrome of soybean. Phytopathology 96:763-770. Online: doi:1094/PHYTO-96-0763.