White mold (Sclerotinia)
Sclerotinia sclerotiorum, S. minor, and S. trifoliorum 1
legumes, sunflowers, canola, most vegetables, tobacco, many flowering bedding plants, and stone fruits
Diseases caused by Sclerotinia spp., generally known as 'white mold', can affect hundreds of plant species including many important crops. The pathogens are favored by cool, moist conditions, but show surprisingly broad ecological distributions.
1 Several other Sclerotinia species cause diseases other than white mold. The pathogen that causes dollar spot of turf historically has been called Sclerotinia homoeocarpa, but is likely to be reclassified as Lanzia or Moellerodiscus.
Symptoms and Signs
White mold is easily identified by the characteristic white cottony mycelium of the pathogen that grows on the surfaces of infected aerial tissues (Fig. 1). The hyphae produce enzymes and oxalic acid, creating water soaked lesions, frequently with a distinct margin. Secondary symptoms such as wilting, bleaching, and shredding also can be observed on above-ground tissues including stems, leaves, petioles and reproductive organs.
At later stages of the disease, the cottony hyphae of the pathogen aggregate into (typically) pea-sized clumps of mycelium (Fig. 2). These clumps eventually mature into hard black sclerotia (Fig. 3), which are most commonly found on the outer surface of the diseased tissue, but sometimes inside of soft host tissues or cavities such as floral receptacles, fruits and the pith of stems.
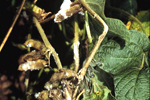 Figure 1 |
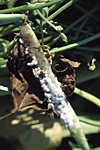 Figure 2 |
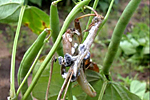 Figure 3 |
Aerial infections
Ascospores of Sclerotinia spp. infect upper portions of plants to cause diseases such as flower blights, stem rots, fruit rots, and head blight. Initially, lesions appear as water-soaked spots that expand irregularly and indeterminately. As the lesions enlarge, affected stems may be girdled (Fig. 4), which causes distal portions of the plant to wilt and then become necrotic (Fig. 5). Affected tissues may develop a soft watery consistency, and a thick white mycelium forms, often both inside and outside the tissue. As the disease progresses, desiccated plant tissues appear bleached (Fig. 6) compared to those senescing normally, and frequently, diseased tissue develops a shredded appearance.
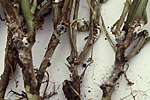 Figure 4 |
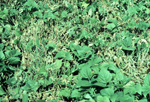 Figure 5 |
 Figure 6 |
Crown rots and basal stem infections
These kinds of infections occur when S. minor, and occasionally S. sclerotiorum, infect plant roots and/or crowns by hyphal, or myceliogenic, germination of soil-borne sclerotia. In sunflower wilt disease, caused by S. sclerotiorum, tissues near the soil line typically show symptoms about the time of flowering; as the infection spreads, a girdling, basal stem canker is formed (Fig. 7) with bleached and shredded pith. In wet weather, fluffy white mycelium forms on the stem surface. The crown and basal stem cankers can cause plant turgor pressure to decrease rapidly, resulting in a sudden wilting of leaves. In ‘drop’ disease of lettuce, infection of roots by S. minor leads to crown infection and girdling of the stem; plants turn brown with a soft watery decay, followed by collapse of the leaves and finally of the whole plant (Fig. 8).
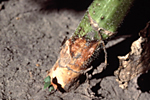 Figure 7 |
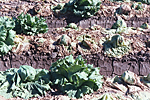 Figure 8 |
Pathogen Biology
Sclerotinia sclerotiorum (Lib.) DeBary, S. minor (Jagger), and S. trifoliorum (Eriks) are necrotrophic fungal pathogens in the Ascomycota, Order Helotiales. All three species produce fluffy white mycelium on and in infected plant parts. This mycelium aggregates itself into sclerotia (Fig. 9, 10), wwhich are the structures that allow Sclerotinia species to survive in soil in the absence of a plant host.
Sclerotia have a hard, black exterior rind with a white to light beige interior. They are irregularly shaped, and typically measure 2 to 5 mm in diameter and up to 25 mm in length for S. sclerotiorum and S. trifoliorum. S.minor forms smaller sclerotia (0.5 to 3 mm). Sclerotia buried in the plow layer of soil can survive and remain infective for up to 5 years.
Carpogenic (apothecial) germination of sclerotia
Sclerotia of Sclerotinia spp. germinate by one of two methods. Sclerotia of S. sclerotiorum and S. trifoliorum most commonly produce a mushroom-like fruiting body termed an apothecium (Fig. 11). At soil depths of up to 2 cm, apothecia can extend from the sclerotia to reach the soil surface. One or several apothecia can emerge from a single sclerotium (Fig. 12). Apothecia are fleshy-colored discs measuring 4 to 8 mm in diameter. They produce ascospores (Fig. 13) as a result of a sexual process, with ascospore morphology differing somewhat between S. sclerotiorum and S. trifoliorum. Each apothecium may release in excess of 10 million ascospores (Fig. 14) over a period of several days, which are blown by wind to the aerial portions of plants. Ascospores are hyaline (clear or non-pigmented), unicellular and thin-walled, and consequently, they survive for only a few days after release.
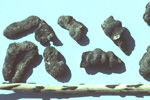 Figure 9 |
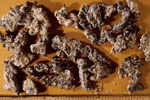 Figure 10 |
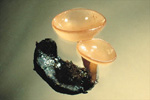 Figure 11 |
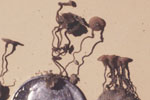 Figure 12 |
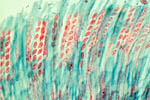 Figure 13 |
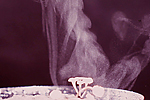 Figure 14 |
No asexual spores (conidia) are produced by Sclerotinia species.
Myceliogenic (hyphal) germination of sclerotia
S. minor sclerotia rarely form apothecia, germinating instead by the direct emergence of hyphae (termed ‘myceliogenic’ or ‘eruptive’ germination). White hyphal strands extend from sclerotia that have been stimulated to germinate by host plant exudates. These hyphae infect roots, and crowns and other plant parts that are touching the ground. Infection then spreads to aboveground plant parts.
Occasionally, S. sclerotiorum germinates myceliogenically rather than carpogenically. In a sunflower crop, this type of germination can result in a serious disease called sunflower wilt (or basal stem rot) (Fig. 7).
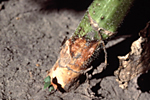 Figure 7 |
Disease Cycle and Epidemiology
Disease Cycle/Epidemiology
Carpogenic germination of sclerotia
Following a period of conditioning in a near-saturated, relatively cool environment (10 to 20°C), soilborne sclerotia of S. sclerotiorum located within 2 cm of the soil surface produce apothecia and ascospores. Most frequently, this occurs after a significant rain or irrigation event, and is aided by a shaded, slow-drying soil surface. Ascospores are forcibly discharged (Fig. 14) and moved by air currents up to susceptible tissue. Prolonged periods of leaf wetness (16 to 48 hours) with temperatures in the range of 12 to 24°C promote ascospore germination and infection. Because the ascospores of S. sclerotiorum lack the energy necessary to infect healthy host tissues directly, a highly susceptible ‘weak’ host tissue, such as flower petals or senescing leaves, is typically the first tissue to become infected. Colonized ‘weak’ tissues then serve as a food base to grow mycelium with sufficient energy to penetrate healthy plant parts. As a consequence, major infection events often coincide with bloom and post-bloom periods, as senescing petals commonly drop and lodge in branch axils or adhere to leaves, petioles, stems and developing fruits. With adequate wetness, mycelium moves from the colonized tissue into the vigorous host tissues of stems, leaves, pods, etc. Later in the disease process, sclerotia form, either on the plant surface or within stems and other plant parts. As the plant or plant part dies, the sclerotia fall to the soil where they can survive for multiple years.
In some cases such as cabbage plants and tobacco seedlings, ascospores of S. sclerotiorum are able to infect plants through wounds. In these cases, mechanical or chemical injury, insect feeding or freezing can increase the incidence and severity of white mold.
Although S. trifoliorum also germinates carpogenically, the disease cycle of alfalfa crown and stem rot is unique. Sclerotia of S. trifoliorum oversummer in soil. In the fall, dropping soil temperatures (10 to 15°C) and increased soil moisture cause the sclerotia to germinate to produce apothecia and ascospores. Ascospores are blown to nearby plants, and under cool wet conditions can infect the leaves directly, forming leaf spots that expand over the winter. A soft rot develops, and if the crop was fall-planted, the seedlings can be killed. When established plants are affected, often only stems wilt and die, while the crowns survive. Mycelium spreads over the plants and down to the soil surface. When host tissue is depleted, or when weather turns warm and dry, sclerotia form on the diseased tissues.
Myceliogenic germination of sclerotia
Hyphal germination of sclerotia occurs when soil is wet and cool; optimal conditions for germination are near-saturated wetness with soil temperatures of 12 to 24°C. These conditions are created by extended rainy periods, or by irrigation events in combination with soil shading due to closure of the crop canopy. Plant infection following this type of germination is favored by similar soil conditions, and is limited to roots, crowns and other plant parts located within 1 to 2 cm of the sclerotia.
Disease epidemiology
White mold is a monocyclic disease, meaning that it has only a primary cycle of infection, and once the host tissue is diseased, it is not contagious (i.e., spore-producing) within the same season. Plant to plant spread of S. sclerotiorum and S. minor may occur occasionally, but this usually is a rare occurrence.
Most severe disease situations in a field develop from sclerotia that are local within the field soil. In the case of carpogenic germination of sclerotia, ascospore releases into the air typically occur at ground level under closed canopies, and consequently, sharp foci of infection are often observed within the field. Nonetheless, infections initiated by wind-disseminated ascospores from another location have been observed occasionally
Introduction of the pathogen from outside sources also may occur by contaminated machinery, mass movement of water, and by wind-blown crop debris. In some hosts, seed may be internally infected with mycelium of Sclerotinia, or inadequately cleaned seed may be contaminated with seed-sized sclerotia. Numerous broadleaf weeds also are hosts of white mold, and may contribute to a persistence of sclerotia within a field.
Sclerotinia species are homothallic (self-fertile), and distinctly clonal populations of
S. sclerotiorum and
S. minor have been found in infested fields. Different clonal groups can occur together, and in some cases, clonal groups have been found to differ in pathogenic aggressiveness.
Disease Management
Management of Sclerotinia occurs at several stages of crop development. Techniques include: making the crop microclimate less conducive for infection, utilization of effective fungicides to protect susceptible plants, elimination of sources of inoculum, and selection of resistant plants. Successful disease control commonly requires implementation and integration of multiple techniques.
Cultural practices that influence crop microclimate
Canopy management. Cropping practices that reduce the intensity and duration of a disease-favorable microclimate within the canopy can lessen white mold severity. Factors that may influence the microclimate include row spacing and orientation, nitrogen fertilizers, and cultivar selection. Studies on row spacing in legume crops consistently show that white mold incidence is lower in crops with wide row widths than those planted in narrow rows. Consequently, the management goal is to space rows at the distance that will maintain plant densities for maximum yield while providing for adequate room to facilitate air movement to reduce high moisture microclimates within the canopy. Precision seeding helps to optimize plant spacing and avoids clumping of plants. Because infection by ascospores of S. sclerotiorum and S. trifoliorum requires an extended period of free moisture, orienting rows parallel to the direction of the prevailing winds also may be of some value in quickly drying the canopy after a rain or irrigation event. In addition, to avoid dense crop canopies, applied nitrogen should not exceed the optimal rate for a particular crop. Lastly, when choices are available, cultivars that mature early and have a more upright, as opposed to a vining (prostrate) growth habit can provide ‘avoidance’ or ‘escape’ resistance, generally resulting in less disease.
A primary cropping practice for prevention of alfalfa crown and stem rot (caused by S. trifoliorum) is to plant seed in the spring or late summer rather than the fall. Warmer temperatures at these times inhibit germination of S. trifoliorum sclerotia; however, planting must be coordinated to ensure adequate soil moisture for the crop.
Sprinkler irrigation. When a crop is irrigated, the goal is to manage irrigation events to reduce the frequencies of 12 to 24 hour periods of leaf wetness, especially during the bloom period, when flower petals can become colonized by the ascospores of S. sclerotiorum. Water deprivation at bloom, however, may compromise the quality of the harvested product. Therefore, a strategy known as ‘irrigation cut-off’ can be used to manipulate the duration of time in a day that foliage remains wet after irrigation. This technique simply requires initiating daily irrigation events in the very early morning and stopping all irrigation near noon. The early afternoon ‘cutoff’ allows time for the sun to dry the foliage before nightfall, thereby helping to avoid prolonged periods of plant wetness.
To reduce disease due to S. minor, hyphal germination of sclerotia can be reduced by allowing the soil surface to dry thoroughly between irrigation events. Each irrigation event must therefore provide sufficient water to allow for a prolonged dry period.
Chemical protection of susceptible plants
Fungicides, applied as protectants before infection, especially during the bloom period, are effective in inhibiting infection by ascospores in fields with a history of white mold, and several registered fungicides are available for this purpose. The number of fungicide applications required for disease control depends on the length of the crop season and the period of time that ‘weak’ tissues (flower petals) are available for colonization by ascospores. A single fungicide application carefully timed during the bloom period may be sufficient in some crops such as snap beans and potato, whereas 2 to 3 applications may be necessary in crops with a longer bloom period, such as lima bean. In order to be effective, it is necessary that fungicides penetrate deep into the canopy to adequately cover the flowers and the places on the plant where the senescing petals might adhere or become lodged.
In lettuce production, fungicides can be used to prevent infection by S. minor, provided that the chemical penetrates and persists on the soil surface to provide a barrier protecting the bottom leaves and stems of the plants. In peanut, a combination of plant pruning and fungicide application was shown to decrease infection by S. minor, possibly because a reduced canopy allowed the chemical to more adequately reach the soil surface.
Management of overseasoning inoculum
Crop rotation with nonhost crops reduces the number of sclerotia in the soil by loss of viability over time. In addition, sclerotia may germinate in the absence of a host crop, but without subsequent host infection, new sclerotia are not returned to the soil and numbers are gradually reduced. Crop rotation is most effective when initiated before white mold becomes a serious disease problem in a field.
Even in the absence of a susceptible crop, sclerotia can remain viable in the plow layer of field soil for up to 5 years. If numbers of sclerotia in a field are low, rotations of 3 to 5 years with a nonhost crop may be sufficient. Once the pathogen is well established in a field, and soil is highly infested with sclerotia, crop rotation may be of less value because of the long survival time of these propagules. Consequently, growers may be faced with the difficult problem of finding and growing an economically viable, non-susceptible rotation crop for more than 5 years.
Crop rotation is further limited by the wide host ranges of Sclerotinia spp. Corn, wheat, and sorghum are nonhost crops of S. sclerotiorum that can be planted in rotation with susceptible crops such as soybean and sunflower. For lettuce infected with S. minor, broccoli grown as a nonhost crop has been shown to reduce the number of sclerotia in the field. Host crops of S. trifoliorum are limited to legumes, so grains and annual forage grasses may be used in crop rotation. A number of annual legumes have been shown to be hosts to Sclerotinia spp., so they should not be used as cover crops.
Biological control. Several fungi have been shown to be parasites of sclerotia of S. sclerotiorum. One of these organisms, Coniothyrium minitans, has been released as a commercial product for suppression of white mold due to S. sclerotiorum. In practice, dried spores of this parasite are sprayed onto pathogen-infested crop debris either at the end of a season, or onto the soil surface before planting. Parasitization of sclerotia has been shown to reduce the number of apothecia formed by S. sclerotiorum. In several studies, soil treatment with C. minitans has lessened white mold severity in the crop that follows the treatment. This treatment is most useful when combined with other components of an integrated disease management program.
Tillage effects on white mold are complex. Although sclerotia can survive in the plow layer for several years, only the sclerotia near the soil surface germinate to produce apothecia and ascospores. Therefore, burying infested residues with a moldboard plow can prevent the apothecial germination of sclerotia. A subsequent plowing in another season, however, can bring these sclerotia back to the surface, and any tillage operation can also contribute to the dispersal of sclerotia. An alternative recommendation to control S. sclerotiorum in a soybean–corn (host-nonhost) rotation has been to follow a diseased soybean crop with the use of no-till in the corn crop. This type of tillage leaves the sclerotia near the surface and promotes their germination under the corn. Consequently, the number of viable sclerotia that survive to the next soybean season is greatly reduced.
Weed control. The host range of S. sclerotiorum is very broad and includes many of the most important broadleaf weeds of cultivated crops: Canada thistle, Jerusalem artichoke, lambsquarters, mustard, nightshade, pigweed, ragweed, shepherd’s purse, sow thistle, velvet leaf and vetch. If these weeds occur in a field, they may provide inoculum for a host crop. When rotating to a nonhost crop, the effect of tillage (or lack of it) on weed control is an additional consideration, as poor suppression of broadleaf weeds may lessen the benefit of crop rotation.
Host resistance
Cultivars able to resist infection by S. sclerotiorum would be a predictable, convenient, and inexpensive option for controlling white mold. Unfortunately, identification and selection of highly effective physiological resistance to this pathogen has proven to be a difficult challenge, especially under a variety of field conditions. As a consequence, commercial cultivars that show high levels of resistance to white mold are relatively uncommon. Some cultivars of common bean, soybean, and other crops have been found that show a low level of physiological resistance but at best this generally has only a partial impact on the overall amount of disease. Biotechnological techniques are being used to create novel types of resistance to diseases caused by Sclerotinia spp. For example, a gene from barley that encodes for oxalate oxidase, an enzyme that degrades oxalic acid, has been transferred into peanut, making it much more resistant to infection by S. minor. Such genetically modified cultivars may become a major means of Sclerotinia disease management in the future.
Significance
Sclerotinia diseases, caused by S. sclerotiorum and to a lesser extent S. minor and S. trifoliorum, affect a wide range of crops. Literature reports of crop losses range from negligible to 100%. Crops where average annual losses from Sclerotinia diseases are thought to exceed 1% include bean, eggplant, lettuce, peanut, potato, soybean, and sunflower. Many of the greatest losses occur in intensive cropping environments where irrigation and optimal nutrition create a lush, dense plant canopy that promotes disease development. White mold is a soilborne disease that can develop into a serious and persistent problem when it becomes established in a field.
Sclerotinia sclerotiorum is geographically cosmopolitan and has a broad ecological distribution, although it is most common in temperate regions. Originally it was thought to occur only in cool, moist areas, but it is now known to occur in semi-arid areas as well. Plants susceptible to this pathogen encompass more than 400 species. Important cultivated plants susceptible to S. sclerotiorum include legumes, sunflowers, canola, most vegetables (e.g. potato, tomato, lettuce, and crucifers), tobacco, many flowering bedding plants, and stone fruits. Corn, sorghum, wheat and other small grains are not susceptible to Sclerotinia spp.
In soybean, S. sclerotiorum causes Sclerotinia stem rot, or simply white mold. Common beans are also very susceptible to white mold. Three distinct diseases of sunflower are caused by S. sclerotiorum: wilt disease produced by a basal stem infection, and head rot and middle stalk rot, both developing from ascospore infections.
Sclerotinia minor is found less commonly than S. sclerotiorum. It causes white mold and watery soft rots of vegetables. On peanut crops, S. minor causes Sclerotinia blight, a serious disease affecting all plant parts. S. minor causes lettuce drop by infecting plant roots, although in some fields aerial infection by S. sclerotiorum ascospores may also contribute to the disease.
Sclerotinia trifoliorum infects forage legumes to cause a white mold crown and stem rot. It can be a serious problem on fall-planted alfalfa. In the U.S. it occurs primarily in the southeastern and eastern states. S. trifoliorum has also been found to cause stem and crown rot of chickpea in Australia and California.
Selected References
Abawi, G.S., and R.G. Grogan. 1979. Epidemiology of diseases caused by Sclerotinia spp. Phytopathology 69:899-904.
Adams, P. B., and W.A. Ayers. 1979. Ecology of Sclerotinia spp. Phytopathology 69:896-899.
Atallah, Z.K., B. Larget, X. Chen, and D.A. Johnson. 2004. High genetic diversity, phenotypic uniformity, and evidence of outcrossing in Sclerotinia sclerotiorum in the Columbia Basin of Washington state. Phytopathology 94:737-742.
Bradley, C.A., R. A. Henson, P.M. Porter, D.G. LeGare, L.E. del Rio, and S.D. Khot. 2006. Response of canola cultivars to Sclerotinia sclerotiorum in controlled and field environments. Plant Disease 90:215-219.
Butzler, T.M., J. Bailey, and M.K. Beute. 1998. Integrated management of Sclerotinia blight in peanut: Utilizing canopy morphology, mechanical pruning, and fungicide timing. Plant Disease 82:1312-1318.
Clarkson, J.P., K. Phelps, J.M. Whipps, C.S. Young, J.A. Smith, and M. Watling. 2007. Forecasting Sclerotinia disease on lettuce: a predictive model for carpogenic germination of Sclerotinia sclerotiorum sclerotia. Phytopathology 97:621-631.
Gerlagh, M., H.M. Goossen-van de Geijn, N.J. Fokkema, and P.F.G. Vereijken. 1999. Long-term biosanitation by application of Coniothyrium minitans on Sclerotinia sclerotiorum-infected crops. Phytopathology 89:141-147.
Hao, J.J., K.V. Subbarao, and J.M. Duniway. 2003. Germination of Sclerotinia minor and S. sclerotiorum sclerotia under various soil moisture and temperature combinations. Phytopathology 93:443-450.
Hao, J.J., K.V. Subbarao, and S.T. Koike. 2003. Effects of broccoli rotation on lettuce drop caused by Sclerotinia minor and on the population density of sclerotia in soil. Plant Disease 87: 159-156.
Hollowell, J.E., B.B. Shew, M.A. Cubeta, and J.W. Wilcut. 2003. Weed species as hosts of Sclerotinia minor in peanut fields. Plant Disease 87:197-199.
Kolkman, J.M. and J.D. Kelly. 2002. Agronomic traits affecting resistance to white mold in common bean. Crop Science 42:693-699.
Livingstone, D.M., J.L. Hampton, P.M. Phipps, and E.A. Grabau. 2005. Enhancing resistance to Sclerotinia minor in peanut by expressing a barley oxalate oxidase gene. Plant Physiology 137:1354–1362.
Mueller, D.S., A.E. Dorrance, R.C. Derksen, E. Ozkan, J.E. Kurle, C.R. Grau, J.M. Gaska, G.L. Hartman, C.A. Bradley, and W.L. Pedersen. 2002. Efficacy of fungicides on Sclerotinia sclerotiorum and their potential for control of Sclerotinia stem rot on soybean. Plant Disease 86:26-31.
Otto-Hanson, L., J.R. Steadman, R. Higgins, and K.M. Eskridge. 2011. Variation in Sclerotinia sclerotiorum bean isolates from multisite resistance screening locations. Plant Disease 95:1370-1377.
Njambere, E.N., W. Chen, C. Frate, B-M. Wu, S.R. Temple, and F.J. Muehlbauer. 2008. Stem and crown rot of chickpea in California caused by Sclerotinia trifoliorum. Plant disease 92:917-922
Nelson, B., and A. Lamey. 2000. Sclerotinia diseases of sunflower. North Dakota State Extension Service Bulletin PP-840.
Pethybridge, S.J., F.S. Hay, and D.H. Gent. 2010. Characterization of the spatiotemporal attributes of Sclerotinia flower blight epidemics in a perennial pyrethrum pathosystem. Plant Disease 94:1305-1313
Subbarao, K.V., S.T. Koike, and J.C. Hubbard. 1996. Effects of deep plowing on the distribution and density of Sclerotinia minor sclerotia and lettuce drop incidence. Plant Disease 80:28-33.
Workneh, P. and X.B. Yang. 2000. Prevalence of Sclerotinia stem rot of soybeans in the north-central United States in relation to tillage, climate, and latitudinal positions. Plant Disease 90:1375-1382.
Wu, B.M. and K.V. Subbarao. 2006. Analyses of lettuce drop incidence and population structure of Sclerotinia sclerotiorum and S. minor. Phytopathology 96:1322-1329.