Updated 2015.
Taro leaf blight
Phytophthora colocasiae
Limited, mainly aroids (Araceae), including Colocasia esculenta (taro, kalo, dasheen) and Alocasia macrorrhiza (giant taro)

Wetland (flooded) taro with only two or three
mature leaves due to taro leaf blight disease.
Symptoms and Signs
Phytophthora colocasiae is primarily a foliar pathogen, but it also affects the petioles and corms of its hosts. The first symptoms on taro (Colocasia esculenta) are small, dark brown flecks or light brown spots on the upper leaf surface (Figure 2). These early spots often occur at the tips and margins of leaves where water accumulates. The spots enlarge rapidly, becoming circular, zonate, and purplish brown to brown in color (Figure 3). On the lower leaf surface, spots have a water-soaked or dry gray appearance and hard globules of plant exudate are sometimes present (Figure 4). As spots increase in size they coalesce and quickly destroy the leaf (Figure 5). In dry weather or on some resistant cultivars, the centers of lesions become papery and fall out, producing a “shot-hole” appearance. Dead leaves often hang on their long petioles like flags (Figure 6).
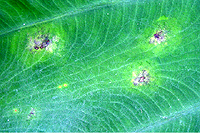 Figure 2 |
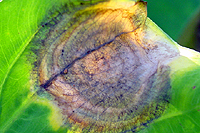 Figure 3 |
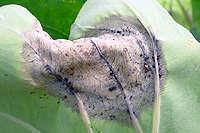 Figure 4 |
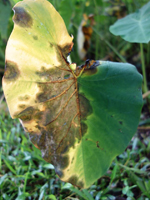 Figure 5 |
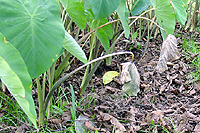 Figure 6 |
Bright orange or reddish brown plant exudate oozing from infection sites is another symptom of leaf blight disease in taro (Figure 7). The presence of yellow tissue around lesions (Figure 8) is not well understood, but could be a cultivar-specific reaction or a stress response to dry weather. Infected corm tissue is brown, firm, and develops rapidly after harvest. A prominent sign of P. colocasiae is the whitish ring of sporangia around the edge of lesions during periods of high relative humidity (Figure 9).
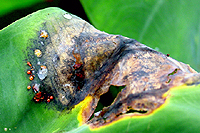 Figure 7 |
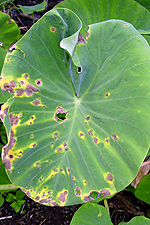 Figure 8 |
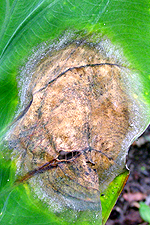 Figure 9 |
Pathogen Biology
The division Oomycota contains many important plant pathogens. Two of its most destructive orders are the downy mildews and the water molds, the latter including Phytophthora and Pythium. Though traditionally taught along with fungi in plant pathology courses, studies indicate that these organisms are more closely related to algae, such as the giant brown kelps. The Oomycota are now placed by most authorities in the kingdom Chromista, though some put them in the kingdom Straminipila (Stramenopila).
Important differences exist between the oomycetes and true fungi. For example, oomycetes have cell walls composed of β-glucans and cellulose rather than chitin. Oomycetes also are unable to produce sterols. These differences can affect isolation, culture, and management of oomycete pathogens. The mycelium of Phytophthora species is composed of tubular hyphae with few or no septa (cross-walls), in contrast to the septate hyphae of fungi. Their nuclei are diploid (2n), compared to haploid (n) or dikaryotic (n + n) nuclei in the mycelia of most fungi.
Asexual reproduction by Phytophthora colocasiae, an aerial species similar to P. infestans, occurs on wet foliage. Sporangia are formed at the ends of short, unbranched or sparingly branched sporangiophores at the margins of lesions. The sporangia are ovoid to ellipsoid with a distinct narrow apical plug (semi-papillate) and average 40 to 50 x 23 µm in size, with a length-to-width ratio of 1.6:1 (Figure 10). Sporangia are usually separated from sporangiophores by rain, leaving a stalk (pedicel) 3 to 10 µm in length attached to their base (Figure 11). During wet conditions, sporangia germinate on the upper surface of leaves and on petioles. When temperatures are cool, near 20°C (68°F), and relative humidity is high (≥90%), most sporangia germinate indirectly (Figure 12), producing zoospores that swim for a few minutes, encyst, and then form germ tubes (Figure 13). This process can occur in two hours or less. In warmer temperatures, up to 30°C (86°F), sporangia can germinate directly and form germ tubes (Figure 14), but under most conditions germination is indirect. The incubation period (time from germ tube penetration of the host to development of symptoms) is two to four days at optimal temperatures of 24 to 27°C (75 to 80°F).
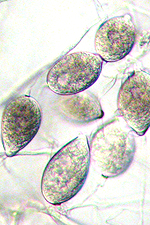 Figure 10 |
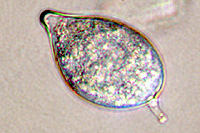 Figure 11 |
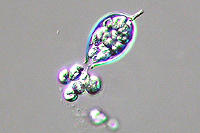 Figure 12 |
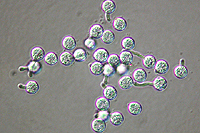 Figure 13 |
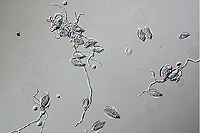 Figure 14 |
Sexual reproduction of this heterothallic species depends on the presence of both A1 and A2 mating types. Hormones produced by one mating type stimulate production of antheridia (male) and oogonia (female) in the opposite mating type. Each antheridium attaches to the base of an oogonium, surrounding its stalk-like attachment (amphigynous) (Figure 15). The nuclei in these organs undergo meiosis and the haploid nucleus from the antheridium unites with a haploid nucleus in the oogonium, forming a diploid oospore 18 to 30 µm (average 23 µm) in diameter (Figure 15).
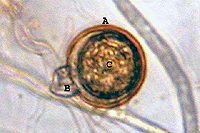 Figure 15 |
Seven “A1A2” isolates of P. colocasiae were found in Taiwan during a survey in 2006 and 2007. These homothallic isolates had the ability to form the male and female reproductive structures needed to produce oospores in the laboratory without an opposite mating type. Interestingly, when haploid zoospores were produced at the end of the sexual cycle (see Disease Cycle below) they were types A1, A2, and A1A2. Isolates of each of these types were able to cause infection and the A1A2 isolate was able to form oospores in plant tissue, as was a combination of A1 and A2 isolates. This finding, though rare, has implications for pathogen survival between crops and for increased genetic variability through sexual recombination.
Phytophthora colocasiae is a warm-weather pathogen, growing most rapidly at temperatures from 27 to 30°C (80 to 86°F). Minimum and maximum temperatures for growth are 10°C (50°F) and 35°C (95°F), respectively. Chlamydospores (thick-walled asexual spores) are sometimes produced in culture and develop either at the end of, or along hyphae. They measure 17 to 38 µm (average 27 µm) in diameter, with walls 2 to 3 µm thick.
Disease Cycle and Epidemiology
Sporangia detach from sporangiophores at the edge of lesions and are spread by rain splash and wind-blown rain. They germinate on leaves and petioles or are washed into the soil where they can infect taro corms.
The pathogen survives as mycelium in plant tissues or as encysted zoospores in soil. If the A1 and A2 mating types or an A1A2 mating type are present, antheridia and oogonia can form and produce oospores. Oospores may act as survival structures in plant tissue or in soil between crops. To date, however, the formation of oospores has been reported only in laboratory trials and not in the field. Infected planting material, along with sporangia and zoospores in wind-driven rain, are the main sources of inoculum in new plantings.
Epidemiology
Sporangia and zoospores are spread by rain splash and wind-blown rain between plants (Figure 16) or on the same plant (Figure 17). Due to the near-vertical growth habit of most taro leaves and their thick, waxy cuticle, many sporangia and zoospores are washed into the soil or splashed onto petioles, often causing them to collapse (Figure 18). Petiole lesions grow rapidly and may produce reddish orange exudates (Figure 19). These lesions should not be confused with the small lesions created by the taro planthopper (Tarophagus proserpina), however, which also produce exudates (Figure 20). In wetland (flooded) taro production, sporangia and zoospores spread between plants and fields in flowing paddy water.
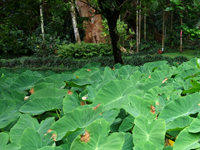 Figure 16 |
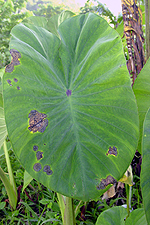 Figure 17 |
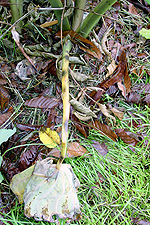 Figure 18 |
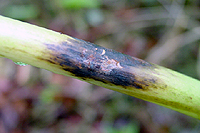 Figure 19 |
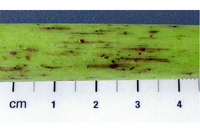 Figure 20 |
The warm, wet climate of the tropics allows taro to be grown throughout the year, ensuring a continuous supply of host plants. Taro is vegetatively propagated and P. colocasiae spreads from field to field and over long distances with diseased planting material. Corms left in the field after harvest can also serve as inoculum sources for newly planted taro plots. Mycelium usually lasts less than 5 days in the soil, but encysted zoospores can survive for up to 3 months. Oospores and chlamydospores are rarely reported in nature, but may act as survival structures in infected plant tissue or in the soil.
Disease Management
Cultural Practices
Early disease management aims at reducing the inoculum level and relative humidity in the field. Taro leaf blight is an explosive disease, however, and cultural and physical control methods are usually ineffective during an epidemic. Roguing (removing all or parts of infected leaves) reduces inoculum levels. As disease severity and roguing intensify, however, the physical removal of leaves mimics the blight by further reducing total leaf surface area.
Field sanitation may decrease inoculum levels early in the season, but sporulating leaf lesions supply enough propagules (sporangia, zoospores) to increase disease. Spacing plants farther apart does little to affect taro leaf blight epidemics. Experiments in both the presence and absence of leaf blight have demonstrated that planting taro closer together improves yield. Close spacing (e.g., 0.5 m or 2 ft) may increase leaf blight severity, but it also increases the total weight and number of corms, though individual corms are smaller.
Chemical Management
Protectant chemical sprays containing copper, manganese, or zinc, have been effective against taro leaf blight, but heavy rains make repeated applications necessary. Acceptable suppression of oomycetes has been reported with systemic pesticides containing either metalaxyl or mono- and di-potassium salts of phosphorous acid. In many countries and island nations, however, taro is a subsistence crop and routine chemical use is neither economically practical nor environmentally or culturally suitable.
Genetic Resistance
Resistant cultivars offer the best long-term solution to managing taro leaf blight. However, desirable cultural characteristics and eating qualities are often lost during conventional breeding. Along with efforts to improve resistance, therefore, breeding programs must also consider improving yield, suckering (desirable for vegetative propagation), taste, texture, and time to maturity.
Isozyme analysis and DNA markers (RAPD) have identified significant genetic differences in isolates of P. colocasiae within and between countries, which may affect the pathogenicity of the isolates. With this in mind, taro from breeding programs should be tested against isolates of P. colocasiae already present in countries before new breeding lines are introduced.
Significance
Taro, Colocasia esculenta, is grown under flooded (wetland, lowland) and non-flooded (dryland, upland) culture on over 1.8 million ha (4.5 million acres) and is the fourteenth most consumed vegetable worldwide. It is an important staple crop throughout the tropics and part of the traditional culture in places like Hawaii and the Samoan Archipelago. The leaves are eaten cooked, and the corm is baked, boiled, fried, pounded into a paste (poi), or made into flour.
Marian Raciborski first reported Phytophthora colocasiae, the cause of taro leaf blight disease, in Java in 1900. From its origin, possibly in eastern India or Indo-Malaysia, it spread throughout the tropical climates of Southeast Asia and the Pacific, Africa, the Caribbean, and the Americas. Reductions in corm yield of 25 to 50% have been reported in the Pacific and 25 to 35% in the Philippines. Losses may be greater among highly susceptible cultivars. Leaf yield losses of 95% were reported for susceptible varieties in Hawaii.
An example of the damage caused by taro leaf blight is the epidemic that occurred in the Samoan Archipelago in 1993 and 1994. In early 1993, the taro export market for the Independent State of Samoa was US$3.5 million, accounting for 58% of Samoa’s exports. By 1994 leaf blight had swept across the islands, substantially reducing taro yield, such that exported taro was valued at less than US$60,000. American Samoa, 90 km (75 miles) to the east, produced 357,000 kg (786,000 lb) of taro in 1993. This dropped to about 22,000 kg (50,000 lb) in 1994 and 5,000 kg (11,000 lb) in 1995. The severity of this epidemic was mainly due to extensive cropping throughout the archipelago with a single, susceptible cultivar.
The warm, humid days and cool, wet nights of the tropics are ideal for reproduction and spread of P. colocasiae. During wet conditions, leaves on taro cultivars that normally live 30 to 40 days may be destroyed by taro leaf blight in less than 20 days. Therefore, a healthy plant that carries 5 to 7 functional leaves may have only 2 or 3 leaves when severely diseased. This reduces net photosynthesis, resulting in a lower corm yield. Highly susceptible cultivars appear to be melting in the field, producing smaller and smaller leaves on shorter and shorter petioles.
Selected References
CAB International, Invasive Species Compendium (literature on taro leaf blight): http://www.cabi.org/isc/search/?q=taro+leaf+blight&page=2&s0=0&s1=0
Cox, P.G., and C. Kasimani. 1990. Effect of taro leaf blight on leaf number. Papua New Guinea Journal of Agriculture, Forestry and Fisheries 35: 43-48.
Erwin, D.C., and O.K. Ribeiro. 1996. Phytophthora Diseases Worldwide. American Phytopathological Society Press, St. Paul, MN.
Gollifer, D.E., and J.F. Brown. 1974. Phytophthora leaf blight of Colocasia esculenta in the British Solomon Islands. Papua New Guinea Agricultural Journal 25: 6-11.
Lebot, V., C. Herail, J. Pardales, M. Prana, M. Thongjiiem, and N.Viet. 2003. Isozyme and RAPD variation among Phytophthora colocasiae isolates from South-east Asia and the Pacific. Plant Pathology 52: 303-313.
Lin, M-J., and W-H. Ko. 2008. Occurrence of isolates of Phytophthora colocasiae in Taiwan with homothallic behavior and its significance. Mycologia 100(5): 727-734 (on-line at http://www.mycologia.org/content/100/5/727.full.pdf+html).
Raciborski, M. 1900. Parasitic algae and fungi, Java. Batavia Bulletin of the New York State Museum 19: 189.
Rossman, A.Y., and Palm, M.E. 2006. Why are Phytophthora and other Oomycota not true Fungi?: http://www.apsnet.org/edcenter/intropp/pathogengroups/pages/oomycetes.aspx.
Trujillo, E.E. 1965. The effect of humidity and temperature on Phytophthora blight of taro. Phytopathology 55: 183-188.
Zhang, K.M., F.C. Zheng, Y.D. Li, P.J. Ann, and W.H. Ko. 1994. Isolates of Phytophthora colocasiae from Hainan Island in China: evidence suggesting an Asian origin of this species. Mycologia 86: 108-112.